TLDR: I redesigned our oxidizer tank to be 50% larger while weighing 7% less. Hydrostatically proof tested to 1,350 PSI.
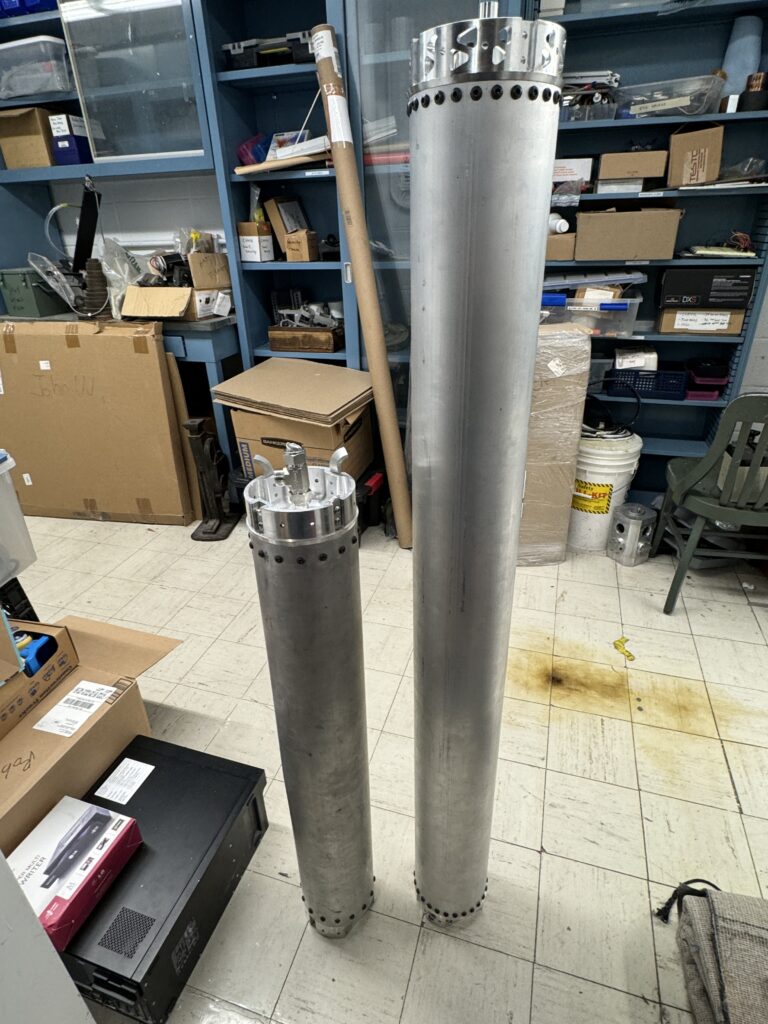
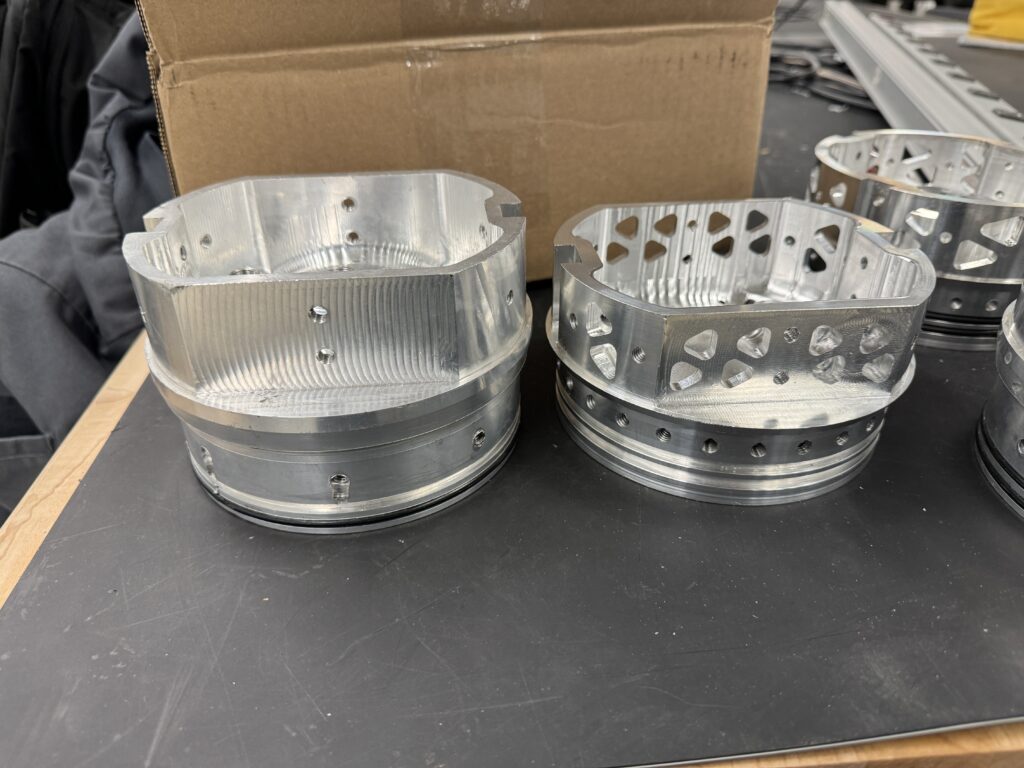
The 2024 ox tank is a substantial iterative improvement on the 2023 oxidizer tank. There were several key areas that I thought could be improved:
- Mass: The 2023 oxidizer tank was redesigned to use a 3/16″ tank wall after hydrostatic failure. Since we were sticking with the same rocket diameter (6″), a 1/8″ tank wall is thick enough to withstand up to 1800 psi without yielding (hoop stress). Therefore, the design tradeoff for using a thicker wall was bearing stress. However, after performing a design optimization analysis, I determined that the added mass of more 1/4-20 bolts was significantly less than using a 0.1875″ tank wall with fewer bolts. So, I changed the tank design to use 26 1/4-20 bolts on each end cap with a 0.125″ tank wall (as opposed to 16 bolts with 0.1875″ wall).
- End Cap Design: For the 2023 oxidizer tank, I used a hemispherical end cap design. The rationale for this choice was that hemispheres minimize wall thickness since they are the most efficient at distributing stress over a given contour. However, I realized that based on the tooling and geometric constraints of the end caps, it would significantly decrease the overall part mass if I used an elliptical, rather than hemispherical, geometry. I think this will be pretty clear in the cross-sections.
- Fill Architecture: In PleaseGoUp, we used a single inlet/outlet port on the bottom end cap. This included a 3/4″ tube stub with Swagelok fittings interfacing to a fill line and feed valve (servo-actuated ball valve). However, this system architecture had a couple of drawbacks. It was significantly heavier than necessary because it used stainless steel tees and reducers, and it added to the overall rocket length. Since the oxidizer tank is now 54″ long and the thrust chamber also increased in length, I must try to minimize the length of the propulsion stack. Keeping this in mind, a marginal increase in machining complexity would pay dividends back in reducing overall vehicle length by 6″ or more.
- Lightweighting: To further reduce component mass, we can remove material from low-load surfaces. On the curved elements of the bulkheads, the load through the retaining bolts of the airframe is relatively low and some mass can be removed.
For the design parameters, I used our internal pressure vessel design spreadsheet that solves for hoop stress, bearing stress, bolt tear-out stress, and casing tensile stress, which were the constraining forces.

The 2023 oxidizer tank used 3/16″ aluminum wall and had 16 1/4-20 bolts. I determined that reducing the wall thickness to 1/8″, which has a hoop stress FOS of 2.04, then increasing the number of bolts from 16 to 26, which gives a bearing stress FOS of 2.06, would result in a lighter overall tank (the bolt mass was second order). Another advantage of using 26 bolts is that the distance between the edge of the tank wall and the bolts can be reduced to 0.375″.
I also decided to change the geometry from hemispherical to elliptical. I used a A=B/2 ellipse, i.e. the radius of the major diameter is the minor diameter, since this was geometrically elegant and well-documented in pressure vessel design. These two cross-sections (2023 vs. 2024 oxidizer tank) show how much material can be removed just with this change:
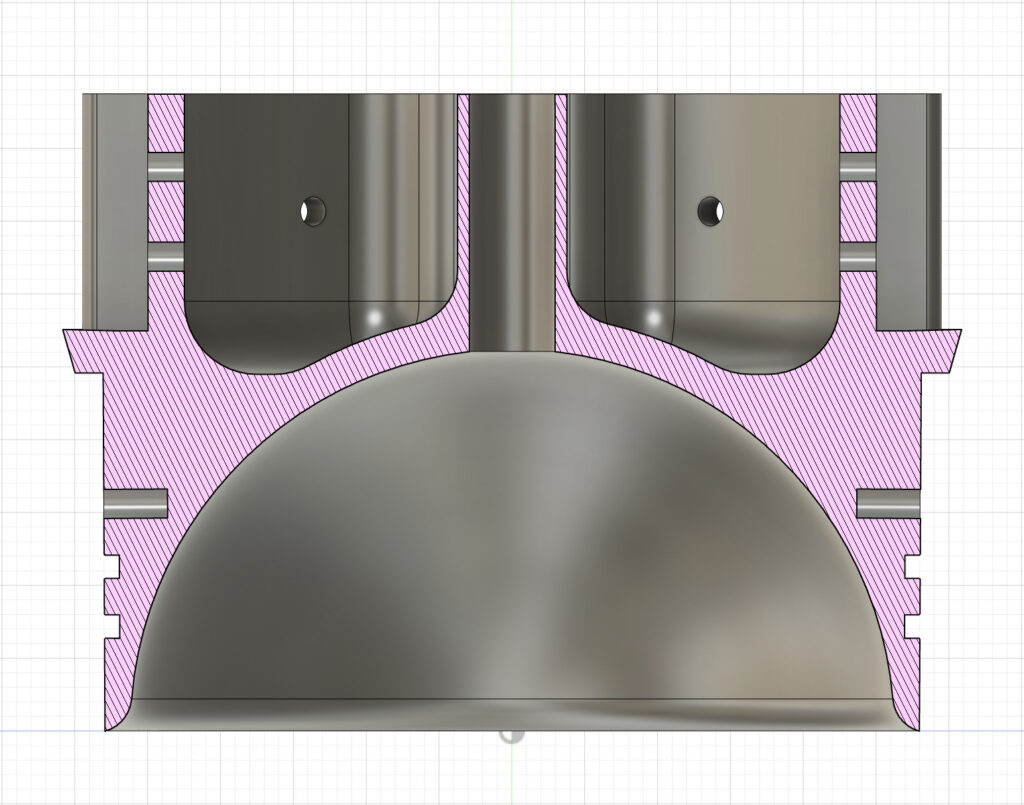
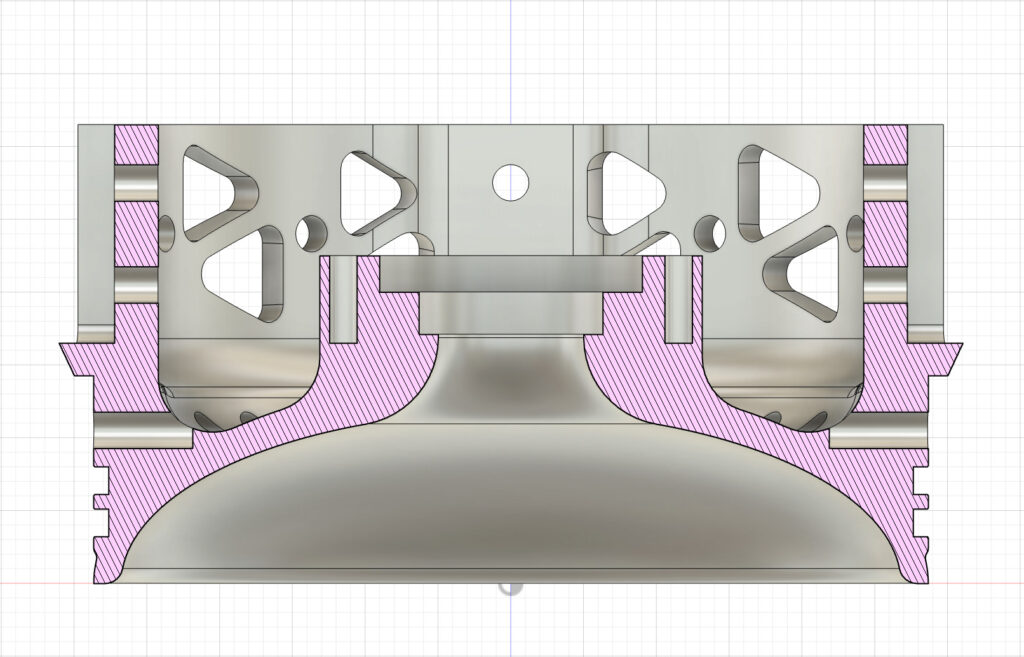
I also added curved lightweight elements to both the top and bottom end caps. It contributed to the overall mass reduction, was a marginal increase in machining complexity (I already needed to run radial operations with a 1/4 flat end mill to clear the strut channels), and made the parts look extra cool.
To size the tank, I used our target oxidizer mass (30.6 lbs), added some margin in case our performance parameters increase during the testing campaign after consulting with our thrust chamber team (35 lbs), then used the maximum temperature (and minimum density) our nitrous oxide would experience (850 psi, 6.066 lbs/gal) [obtained using REFPROP] to size the tank.
This is exactly a 50% increase in length compared to PleaseGoUp, however, the return to a 1/8″ tank wall means the oxidizer tank tube weighs the same. Including the redesigned end caps, the new oxidizer tank weighed 17.3 lbs, compared to the PleaseGoUp oxidizer tank which weighed 18.5 lbs. The tank had a 50% increase in volume while decreasing 7% in total mass.
To summarize, I now have some of the critical dimensions for this pressure vessel (bolted closure design):
- 6″ tank OD, 5.75″ tank ID, 0.125″ wall thickness
- 26 radial 1/4-20 bolts per end cap
- Hemi-ellipsoidal geometry
- Redundant 254 static o-ring seals
For the remaining parameters, consideration of the full fluids system (valve, venting, and integration) were necessary.
The first of these considerations is integration with the pneumatic valve. I decided to use piston seals rather than face seals for the interface because it allowed me to build in 60 thou of axial slip. This gives us some leeway in machining the struts such that no load is transferred through the valve (although I have run FEAs of this edge case and it should be able to support the entire thrust of the rocket unilaterally).
In addition to pneumatic valve integration, as discussed earlier I decided to change the configuration such that nitrous load flowed through a separate radially offset NPT fitting. I also added an additional port for a pressure transducer on the bottom end cap. This serves several purposes:
- Provides redundant tank pressure data ( + pgh of filled nitrous oxide which can fluctuate based on mass and temperature but is on the order of 7.5 psi).
- Provides a direct pressure drop across the pneumatic valve (we also have a transducer at the injector manifold).
- Also allows us to observe any changes in the compressibility effects.
The bottom-end cap design is shown below:
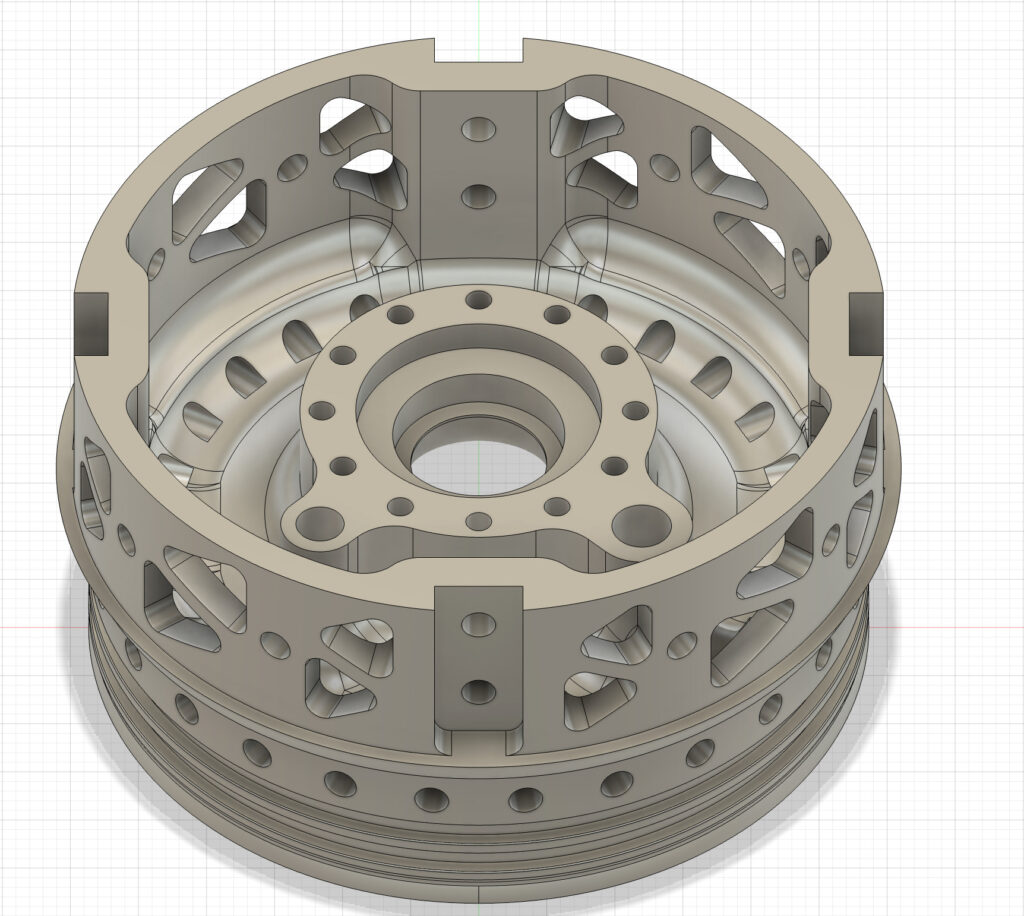
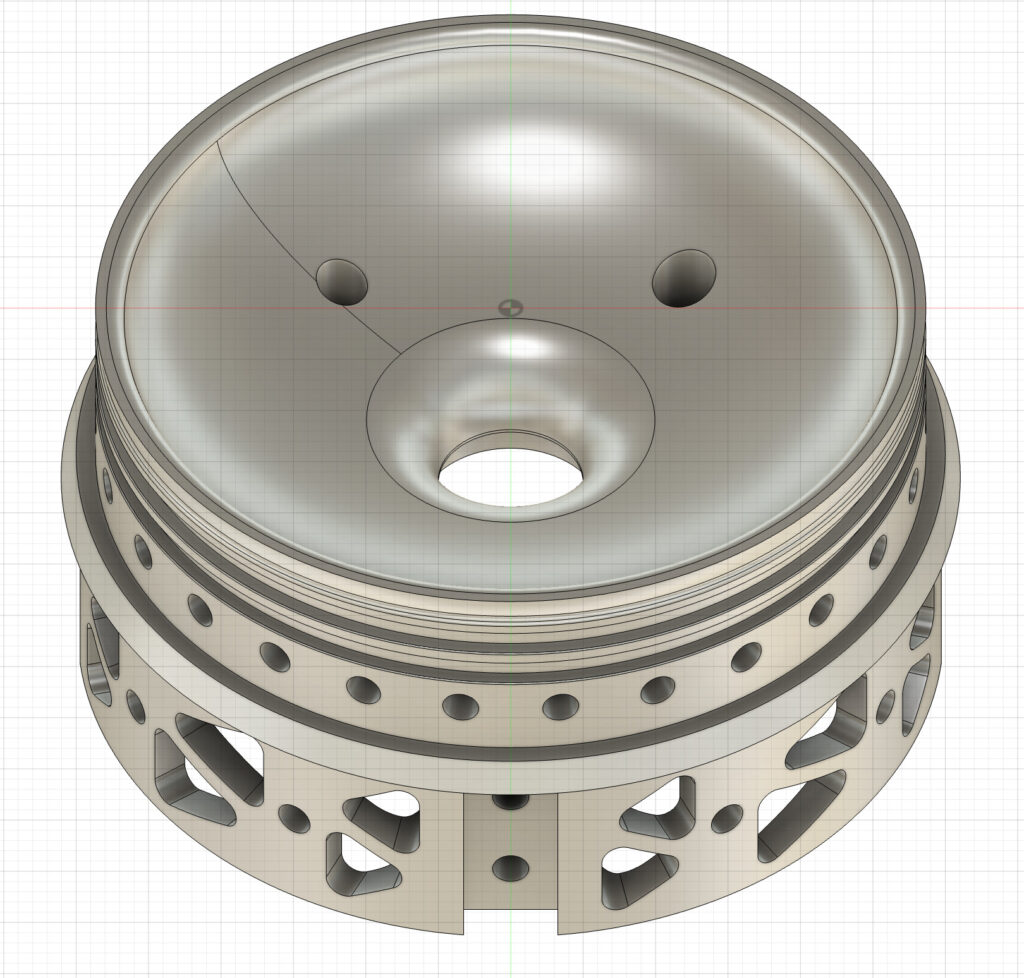
The top end cap was also modified to be easier to integrate. One of the lessons I learned trying to assemble the fitting last year was that adequately tightening fittings was remarkably difficult with the bulkhead rails. So, I radially offset the pressure transducer and venting 1/4 NPT from the center to make this easier.
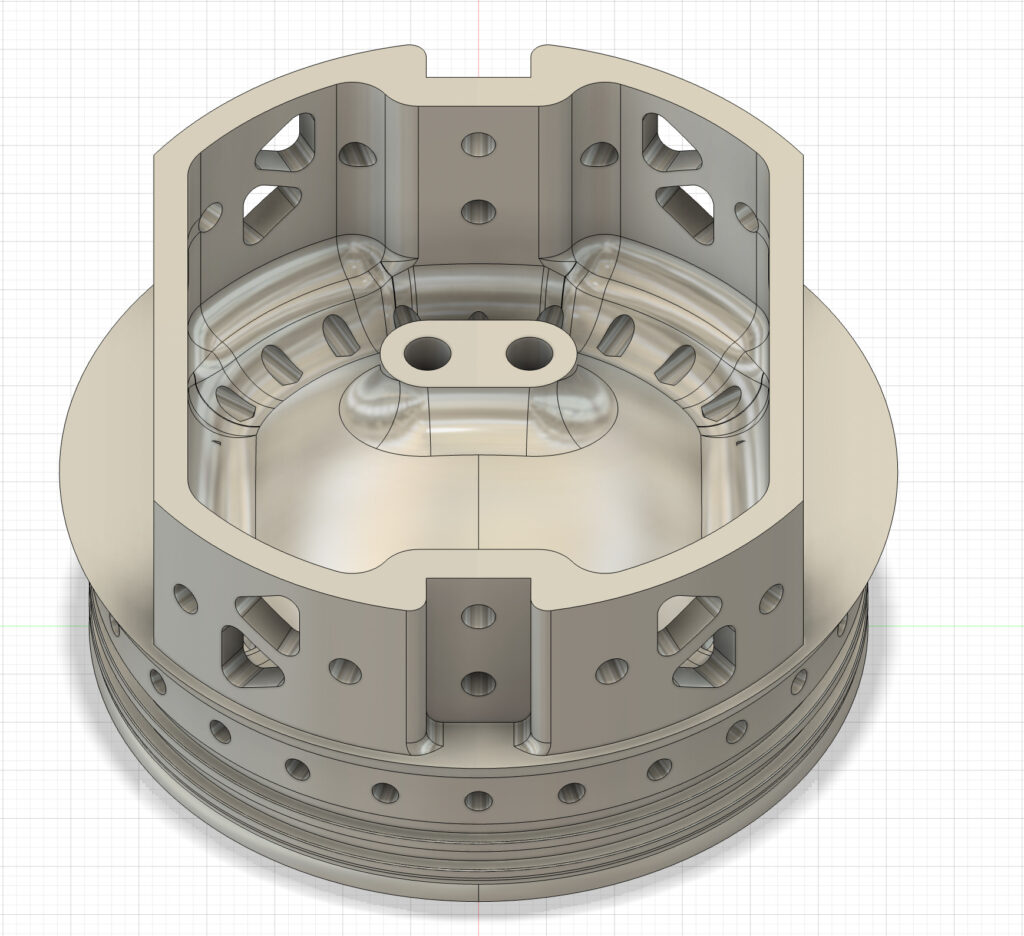
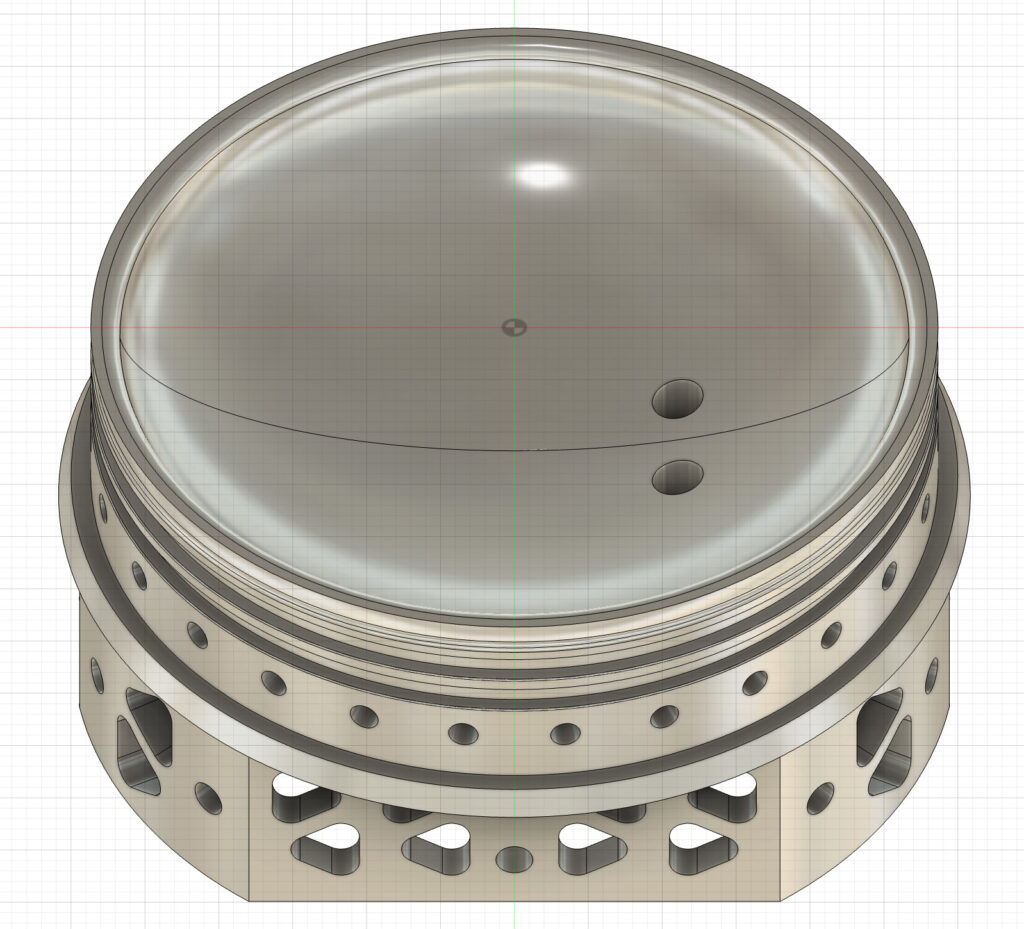
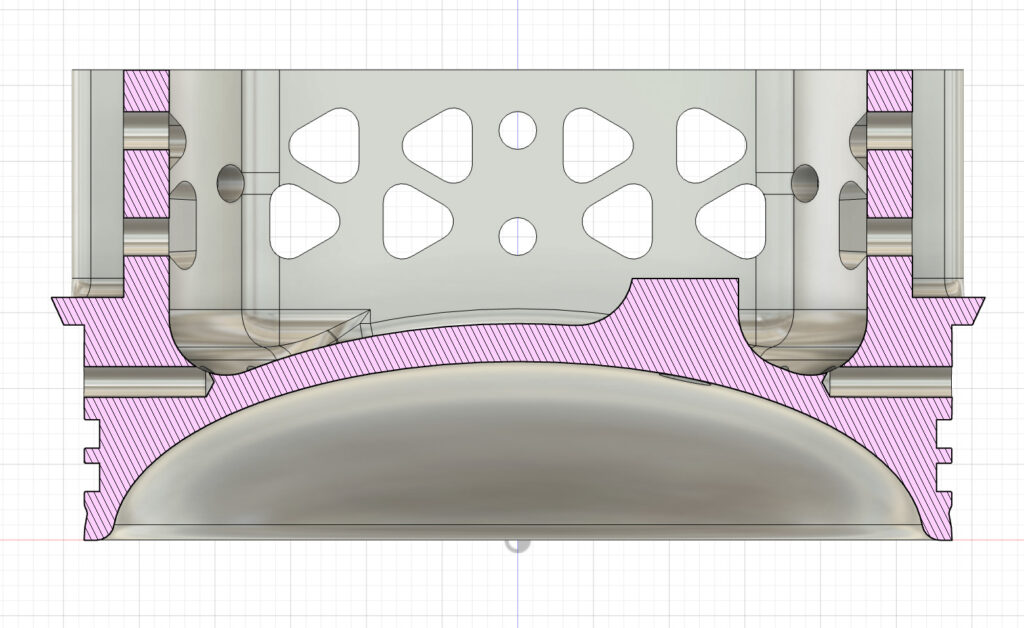
These parts were designed to be machined on our Haas ST-20Y 4-axis CNC lathe. A sped-up video of the CAM of these components is shown below:
After recalibrating the Haas ST-20Y lathe with correct parameters, we went on to successfully machine the top end cap:
Power tapping! It was challenging to get quality footage of other operations due to the coolant spray blocking the windows (we used coolant for the rest of the tapping as well).
The final product:
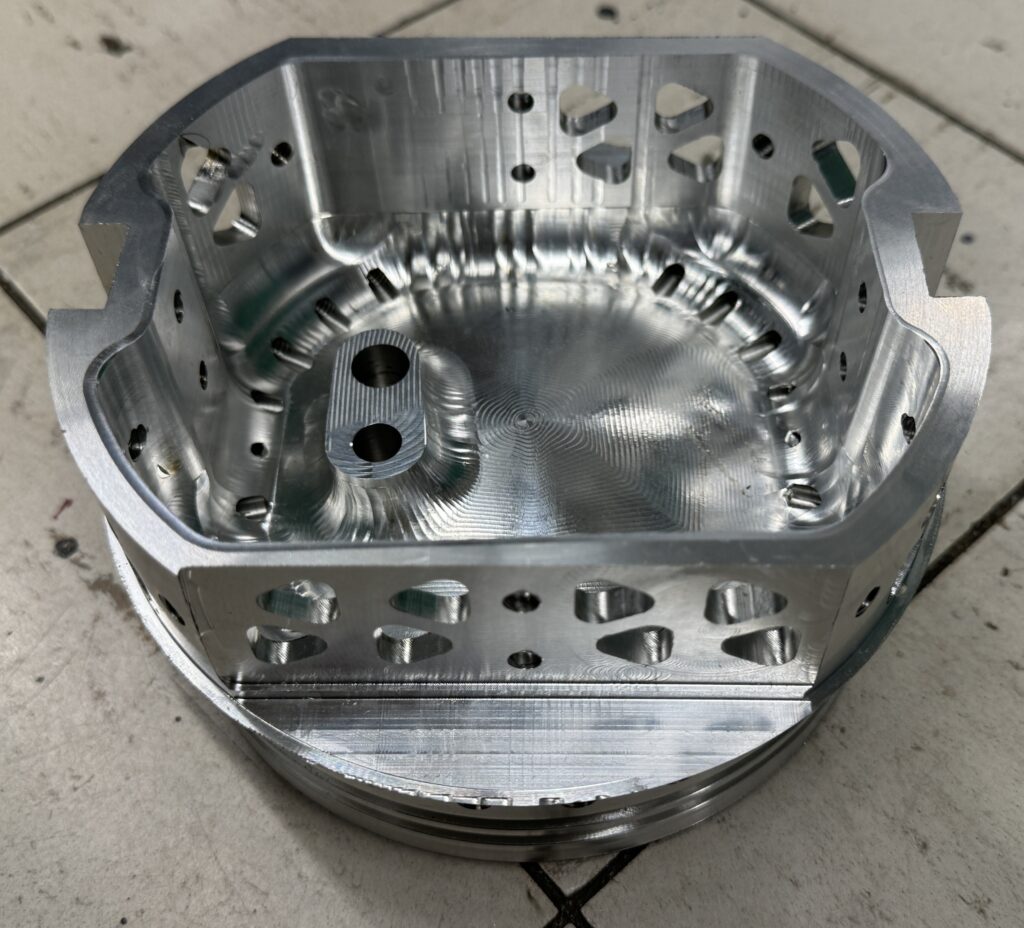
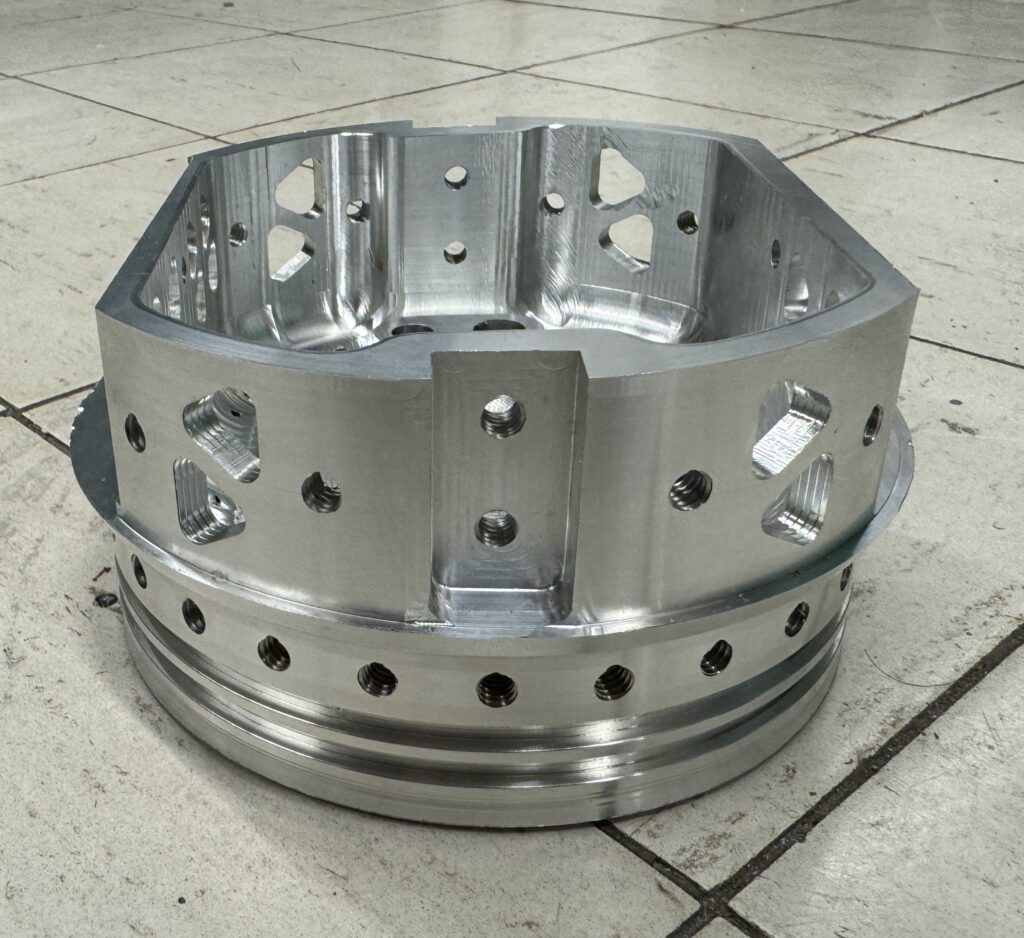
Overall I am extremely pleased with the finish on this part and I think the machining is significantly better compared to the first set of end caps, however, there was one glaring issue with this part: The inner feature had a rotation of roughly 1.5 degrees.
The inner rotation was most likely an artifact of the machine and was not immediately clear. To find the root cause we ran several test parts:
We also performed other diagnostics to determine tram in the X, Y axes.
In this diagnostic, we observed some waviness in the feature (around 4 thou) which is likely due to a bent rail from some past crash (this machine has been through a lot over the years).
We managed to dial in the lathe to extremely tight tolerances, however the axial milling offset persisted and is still a mystery. If you’re reading this and you think you know why, please shoot me an email at aka2205@columbia.edu!
At this point, we had run into finals week and couldn’t continue work, but I decided as a workaround it is probably best to just perform turning and radial milling operations on the lathe, use a custom nylon jig to work hold the bottom end cap, then use the 3-axis Haas Mini-Mill to perform the axial clears.
Returning from the break, I led the team in machining the bottom end cap on the 4-axis mill turn lathe and subsequent workholding for a 3-axis mill.
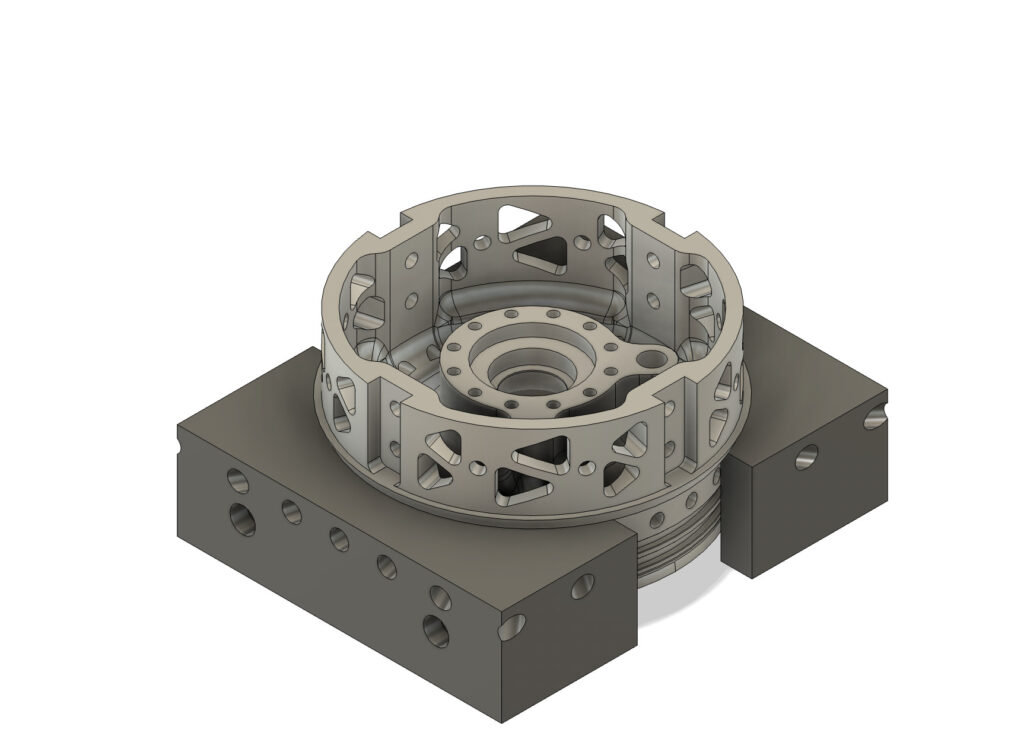
This jig was 3D printed out of Nylon and was exceedingly strong. The CAM for the bottom end cap was very similar to that of the top end cap.
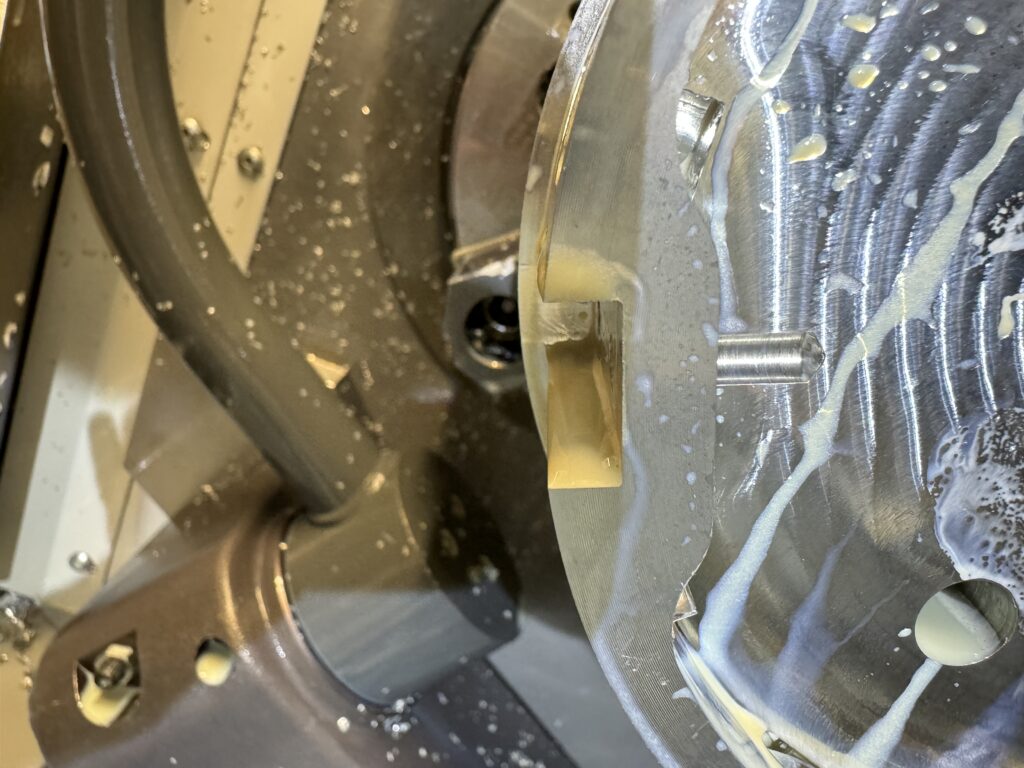
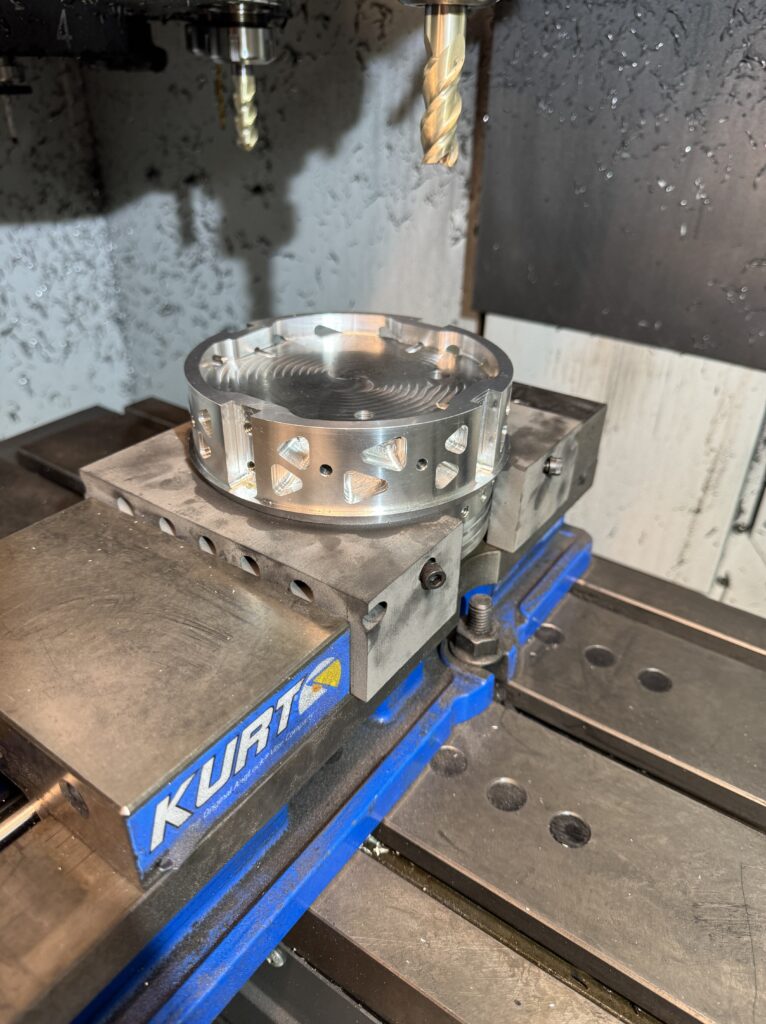
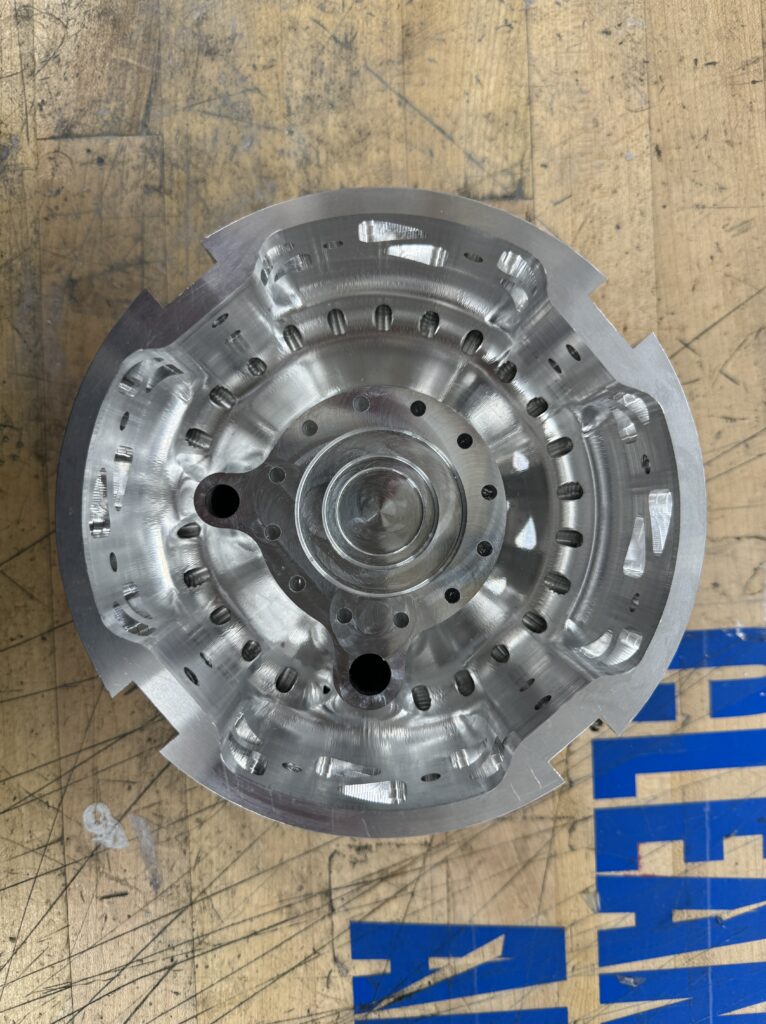
Next up, milling the elliptical geometry for mass savings:
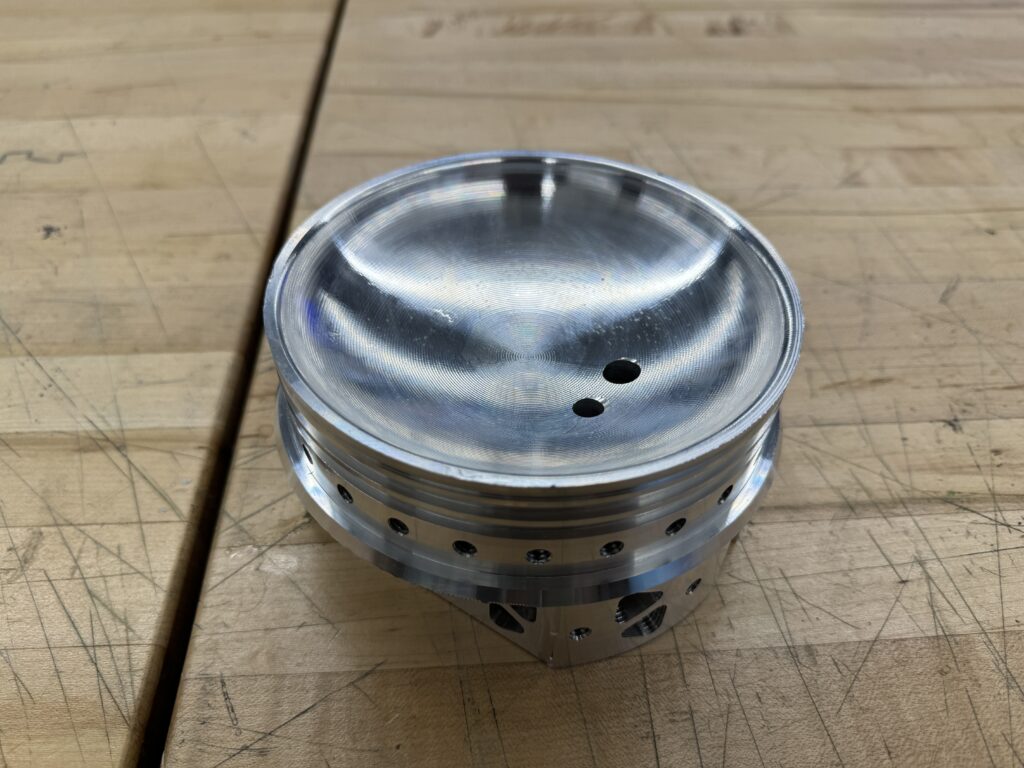
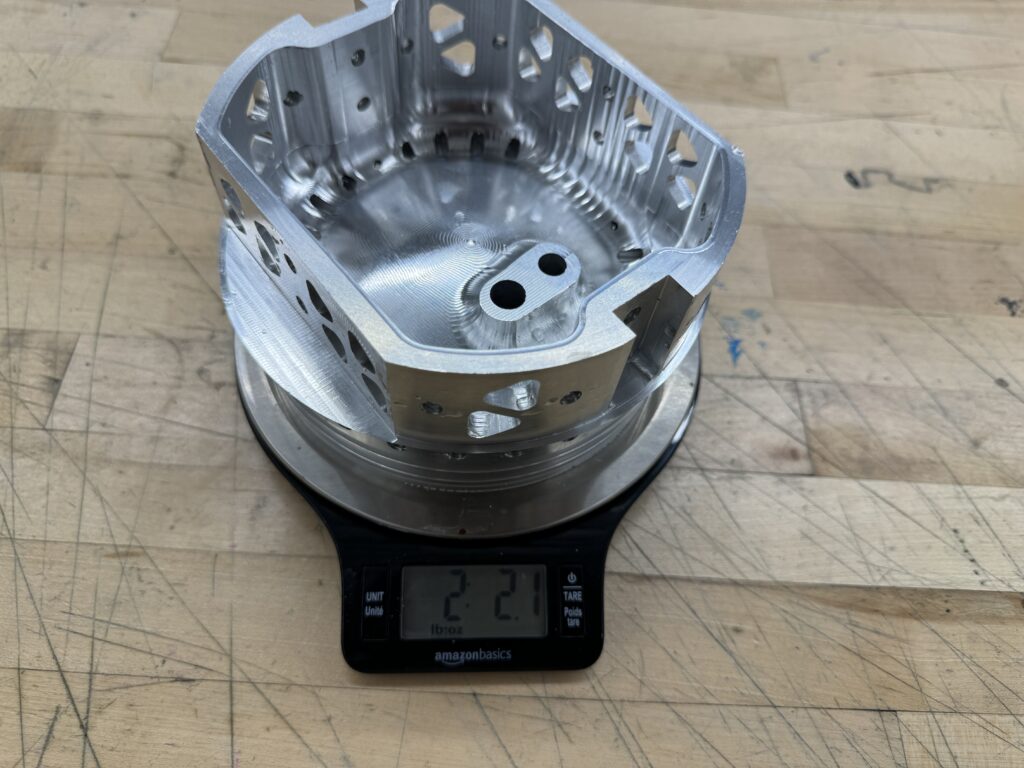
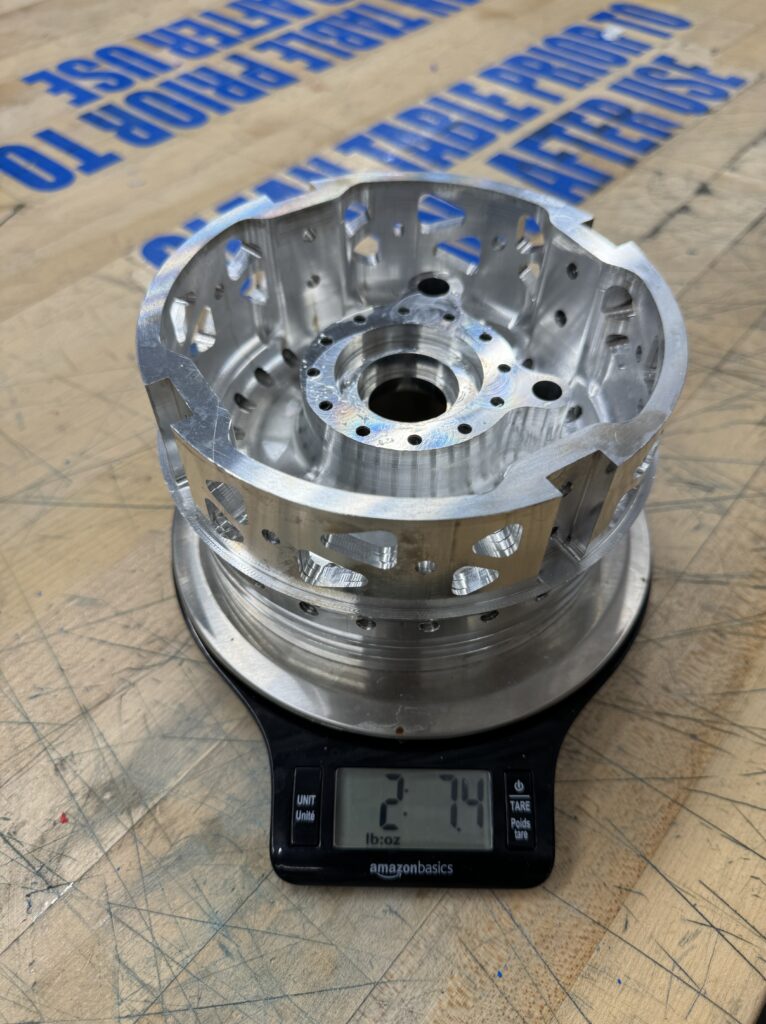
Both parts were remarkably light! The last part to be machined was the flange adapter. This part serves two purposes: seal the bottom end cap for hydrostatic testing and work as an adapter for the ball valve is the situation that we static fire before the pneumatic valve is ready.
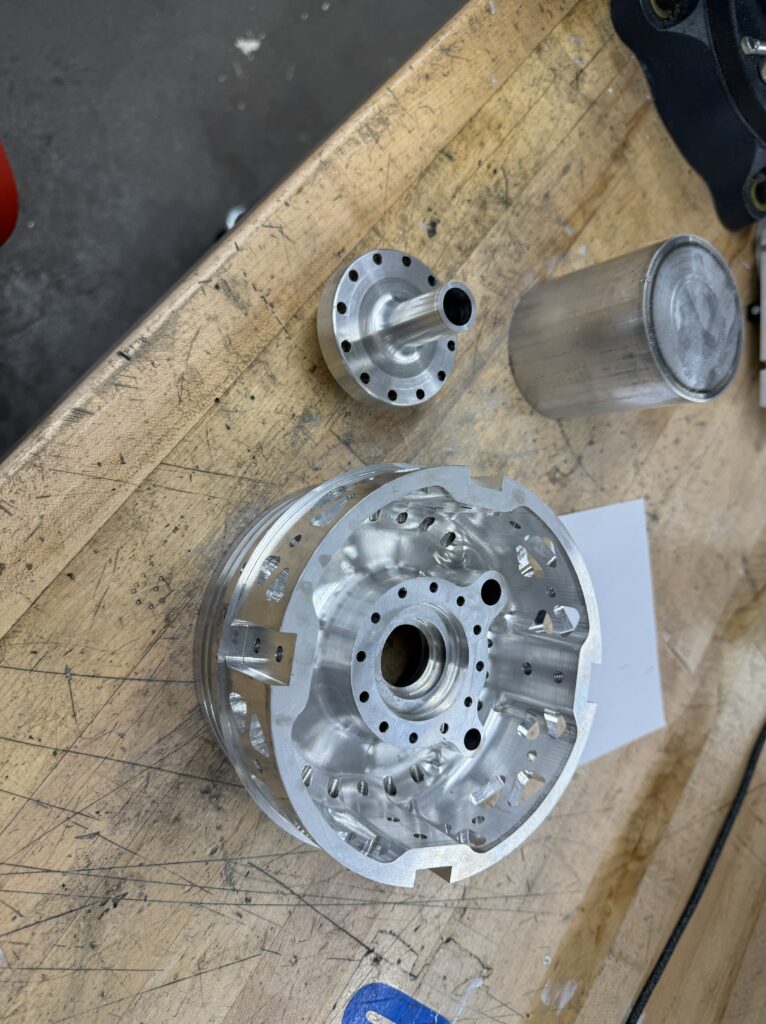
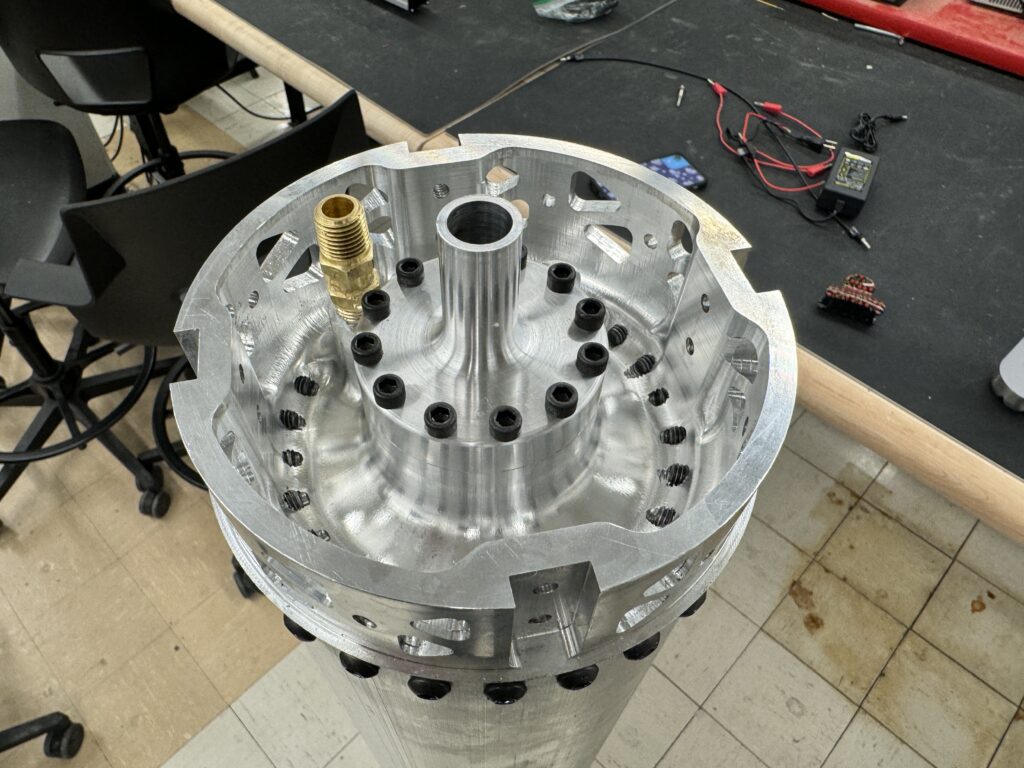
The flanged adapter integrated with the bottom end cap and produced a stunning part. The last task was integrating everything together. To index the 26 holes on the oxidizer tank tube, I used the CNC lathe. The length of the stock was really stretching its abilities, but it was necessary to align the holes.
One change I made was switching from 70D to 90D 254 o-rings for sealing between the end cap and the ox tank tube. The main benefit of this change is improved resilience during installation and higher resistance to extrusion during testing. The final assembled tank and the 2023 oxidizer tank recovered from ballistic impact last June are shown side-by-side.
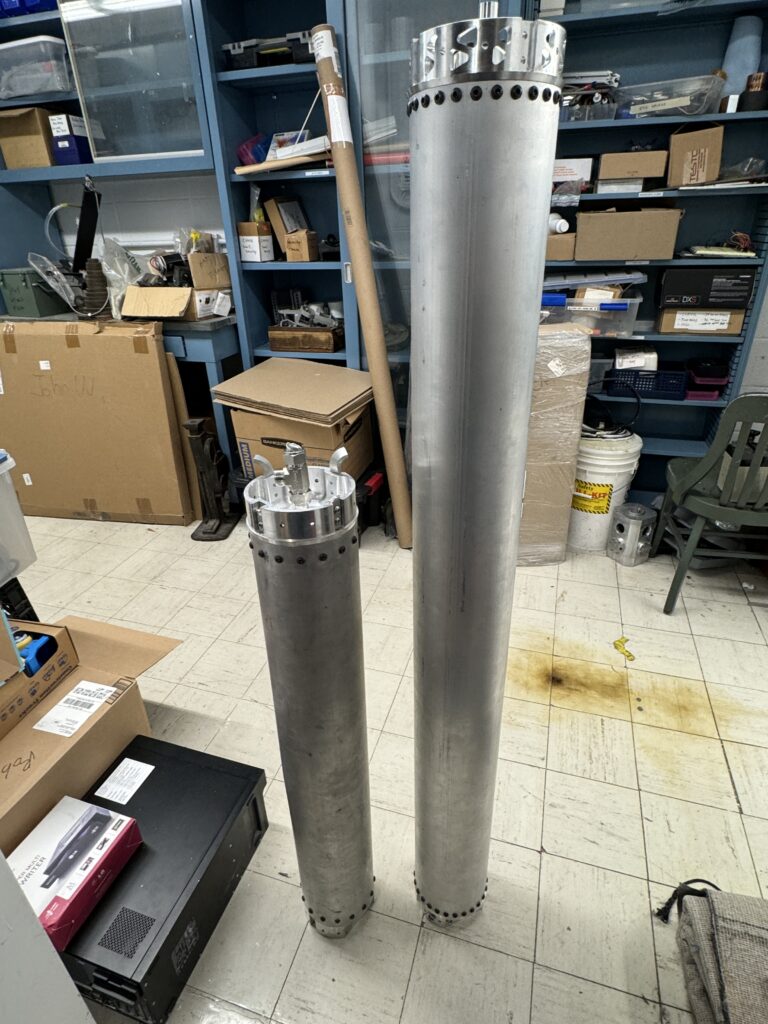
We proof tested the oxidizer tank using hydrostatic testing to 1.5x MEOP for 30 minutes.
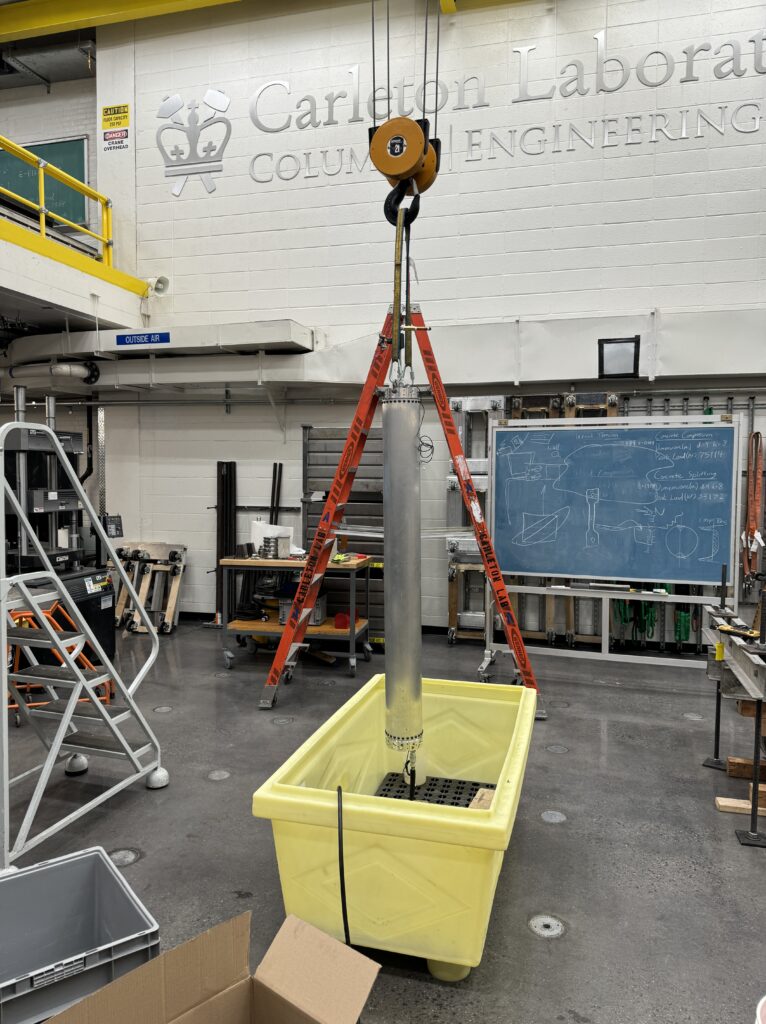
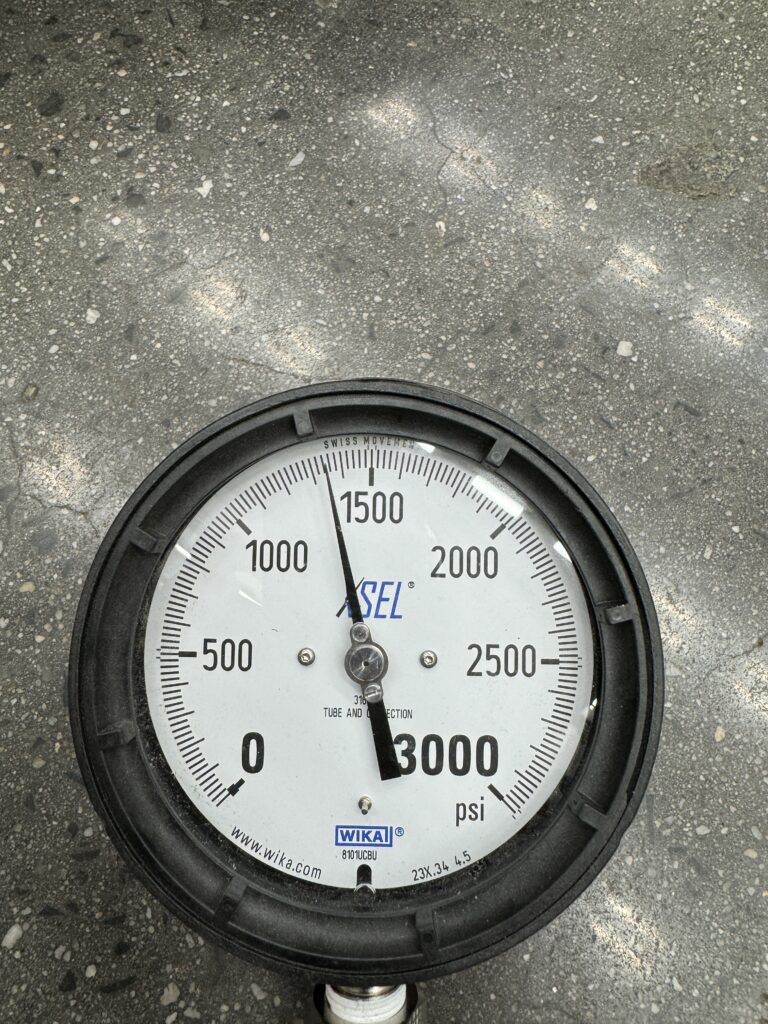
After holding pressure for 30 minutes, it dropped to 1,250 psi. This indicates that there were small leaks, which we confirmed visually at the air release ball valve and pressure transducer crush washers as small drips. We remedied these leaks in future hydrostatic tests with the coaxial pneumatic valve.
This was a huge success and I’m extremely happy with the results. The 2024 ox tank was the most challenging project that I’ve been responsible for thus far, and I’m elated that not only was I able to make design optimizations to reduce mass, but that every hand calc and FEA I ran was validated in hydrostatic testing, while the final product will measurably improve fluids system performance for launch at FAR-OUT.