Summary:
Article:
For the 23-24 rocket development cycle, I led our team in designing, machining, qualifying, and testing a coaxial pneumatic valve. It powered a 1200 lbf hybrid rocket motor, generating 16Gs of acceleration and recovering successfully in June 2024.
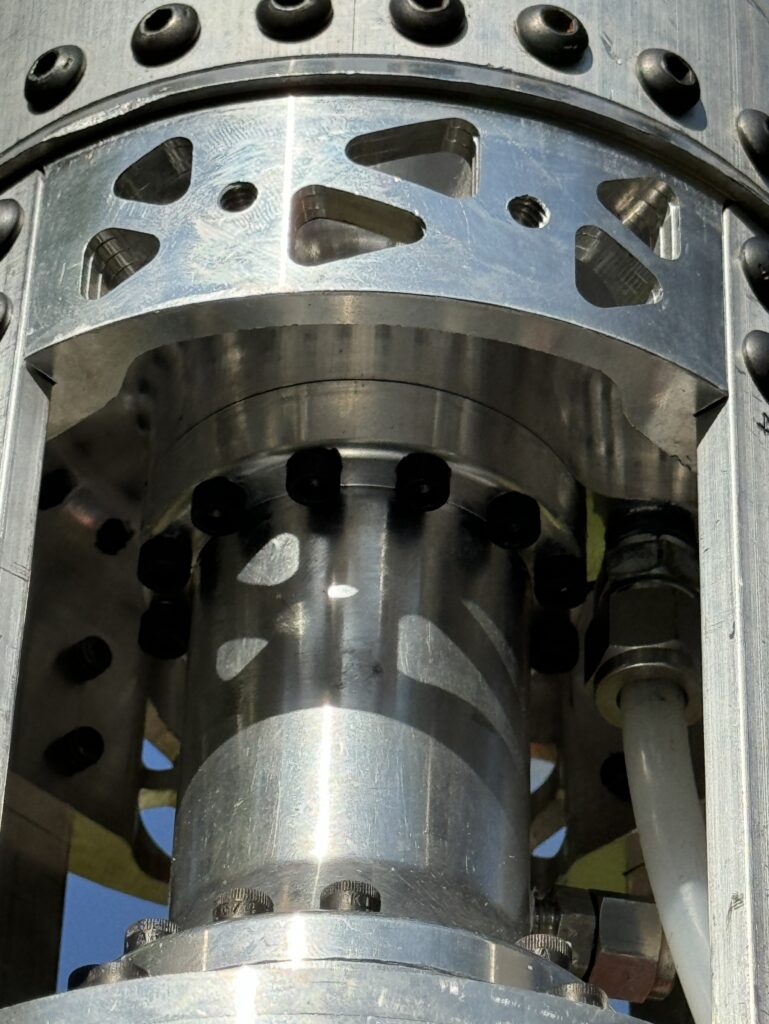
The coaxial pneumatic valve is a pressure-assisted design, with a MEOP of 900 psi and proof of 1,350 psi. It is sealed with 25% glass-filled PTFE, has a 1″ diameter orifice, and has both single and double-acting modes. The valve body is machined from 6061-T6 aluminum. It uses nitrous vapor as the working gas to actuate the valve, with compressed air used to actuate the valve closed after a test.
There were several reasons for developing a custom valve:
- Overall system mass: 316 SS ball valve + 125 kg/cm servo, housing, and linkage added significant system mass to the fluids system. A custom aluminum valve decreased overall system mass.
- Flow Rate: The Swagelok 3/4″ ball valve we used in the 2022/23 rocketry design cycle had an orifice diameter of 0.4″. This had a calculated headloss of 49.21 psi, which significantly dampens blowdown performance and promotes combustion instabilities.
- Actuation Time: The ball valve actuation mechanism was a delicate dance between packing strength and actuation speed. The more torque applied to the packing nut, the higher the friction experienced by the ball and the slower the actuation. To minimize transients in the thrust chamber, the valve should open as quickly as possible.
A coaxial pneumatic valve design was chosen for several reasons. (a) it is manufacturable on the available CNC machines (ST-20Y 4-axis), (b) it has a compact design that leaves plenty of room for additional hardware in the interstage, and (c) similar designs have been implemented by other rocketry teams successfully.
Working Principle:
Step 1: The valve is sealed under static pressure during fill. The spring provides 12.6 lbf of pressure on the PTFE seat. It is pressure-assisted, so sealing improves as tank pressure increases.
Step 2: A pilot valve flows nitrous oxide vapor from the fluids system venting loop into a pressurized volume.
Step 3: The PTFE seal is broken and liquid nitrous oxide flows freely from the tank, around the valve seat, and into the injector. After the tank is drained fully, the pilot valve also purges any high-pressure vapor.

I authored this document explaining all of the calculations used to size the pneumatic valve. It uses a deprecated design with compressed air for actuation, in an updated design the diameter was reduced and valve stop redesigned to account for the new force balance from nitrous vapor pressure.
This is a screenshot of the code I wrote to find the valve actuation time:
The second iteration of this code has more comprehensive models for the o-ring friction, actuation gas pressure, and valve motion. This is a copy of the new code:
Based on all of these parameters, I finalized the dimensions and allocated CAD of components to our subteam members. I instructed them in using the Parker ORD handbook for GD&T of o-ring grooves (dynamic & static), proper CAD modeling techniques in Fusion 360, and FEA of components to validate a suitable factor of safety. I modeled any remaining components myself, then instructed our team in computer-aided machining (CAM).
I created this drawing packet summarizing each in-house component.
Machining the pneumatic valve was a joint effort. Due to tolerance stack-ups in the manufacturing process, we scrapped some components or modified them.
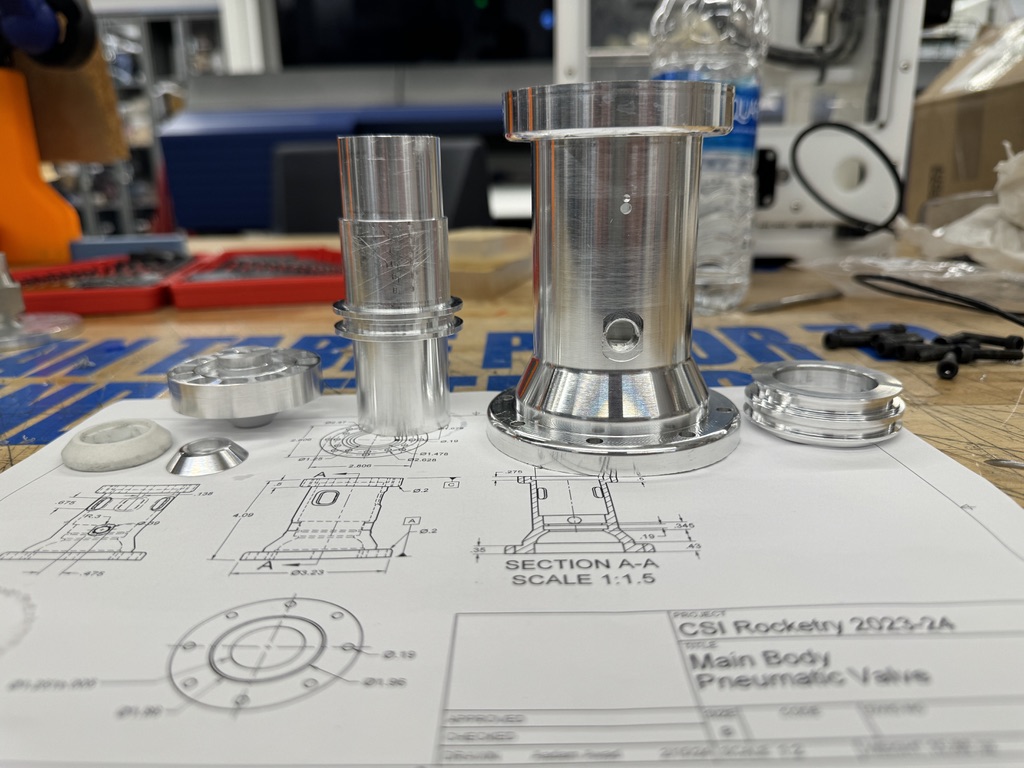
After completing valve machining, several iterations of the PTFE seal geometry were required. Initial hydrostatic testing showed significant leakage through the PTFE seal, indicating the piston was not compressing the PTFE sufficiently.
After three PTFE seal iterations, the leak path was identified as being a coaxial tolerance stack-up in the seal seat design. I designed the seal seat outer lip to be the same diameter as the piston erroneously. This meant that any loss in concentricity would lead to a part of the piston contacting aluminum, rather than PTFE, and sealing insufficiently. The fix for this was re-machining the PTFE ring with a raised inside lip, which contacted the piston first. This worked exceptionally well and there have been no sealing problems since.

The coaxial pneumatic valve enabled as-designed oxidizer mass flow rates to the 1200lbf Valkyrie 2 hybrid rocket motor. The increased combustion chamber temperatures and pressures led to a burn-through and required redesigning the phenolic insulation.
Another benefit of the coaxial pneumatic valve was a significant reduction in fluid interstage length:


A:B of valve configurations.
Finally, the coaxial pneumatic valve powered our hybrid rocket engine at the inaugural FAR-OUT competition in June 2024.