Summary:
I set a personal goal to certify NAR L1 and L2 during the summer of 2023. After scratch-building a high-powered rocket with a modular motor interface, I accomplished my goal and earned the certifications.
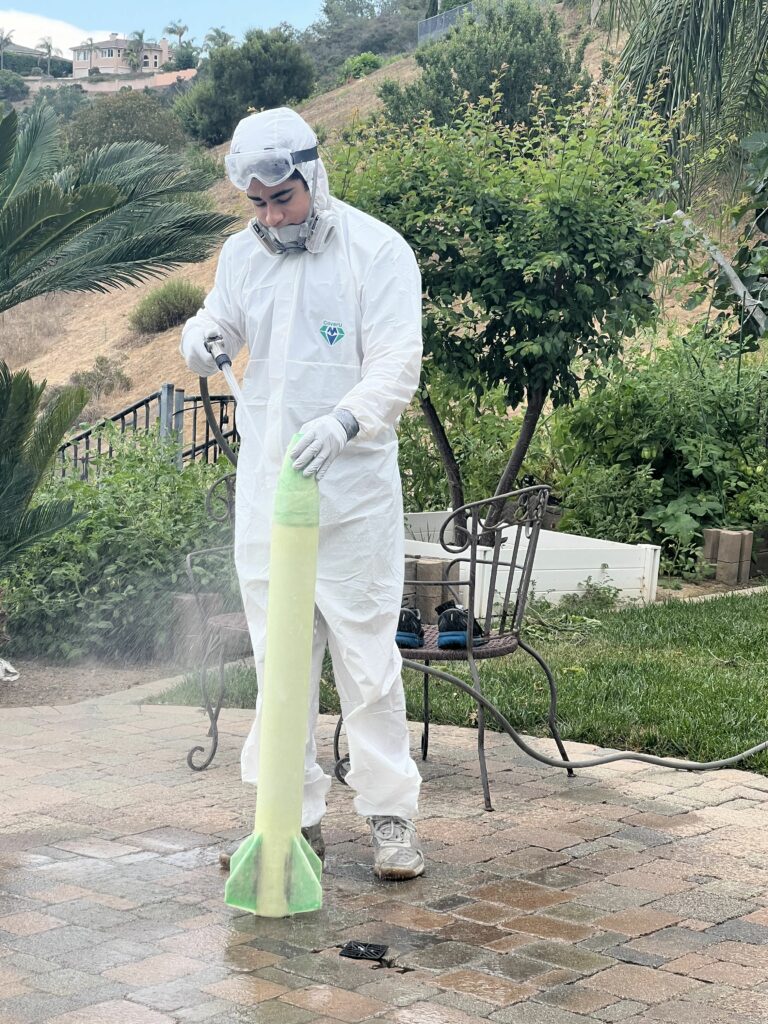
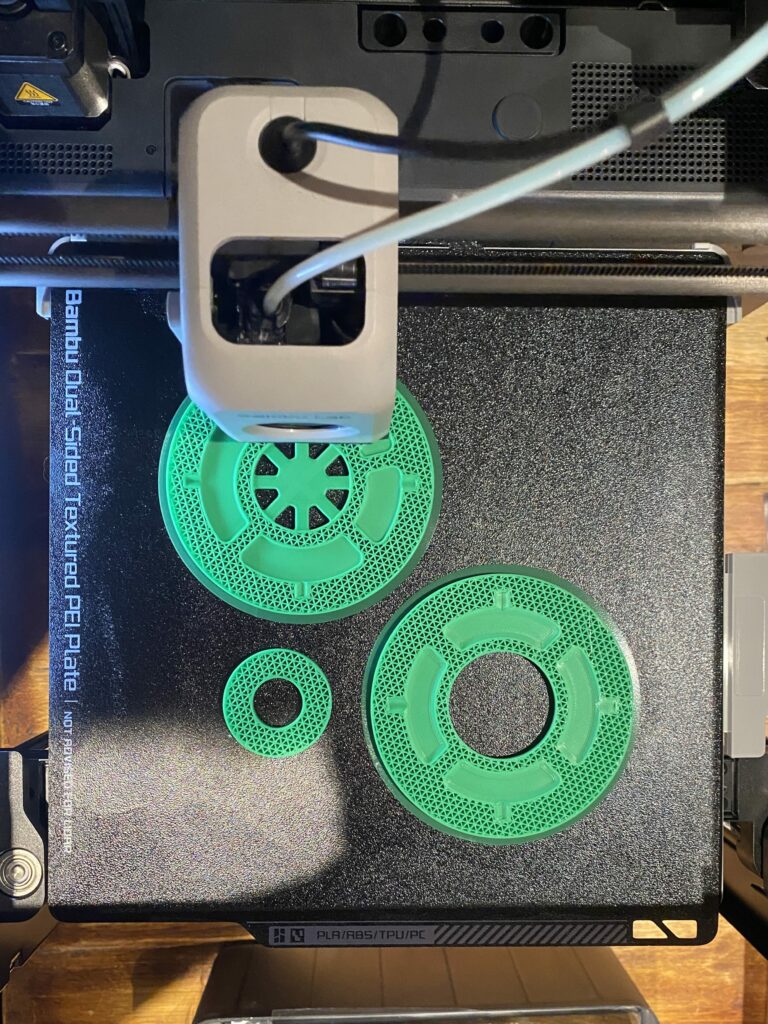
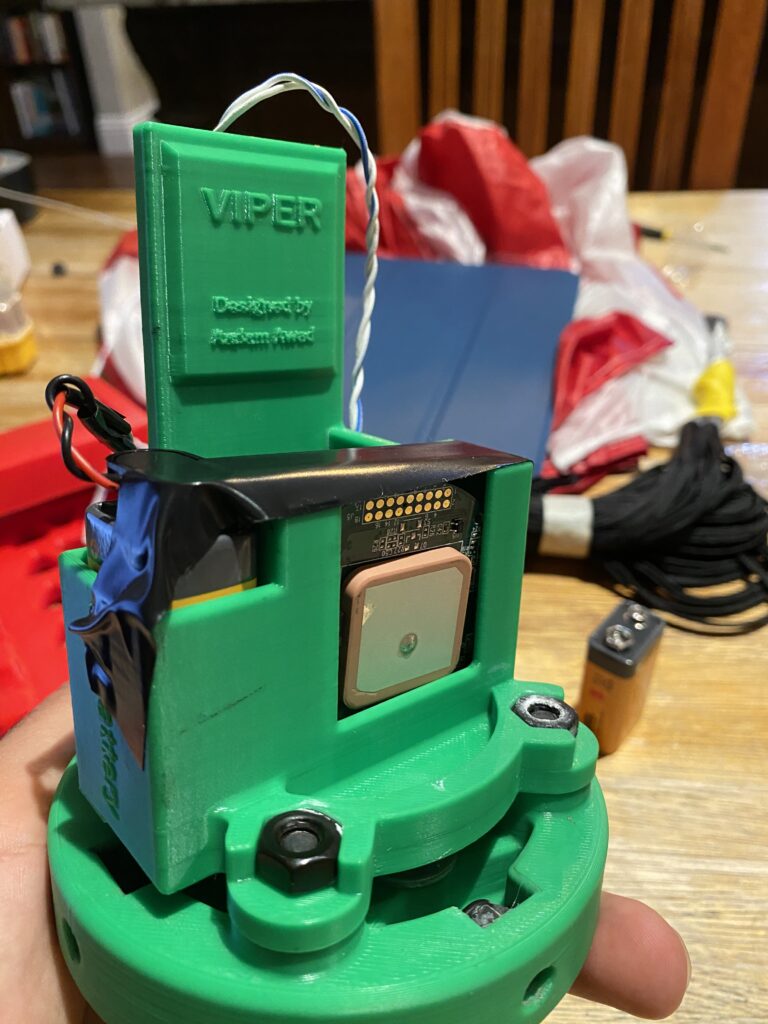
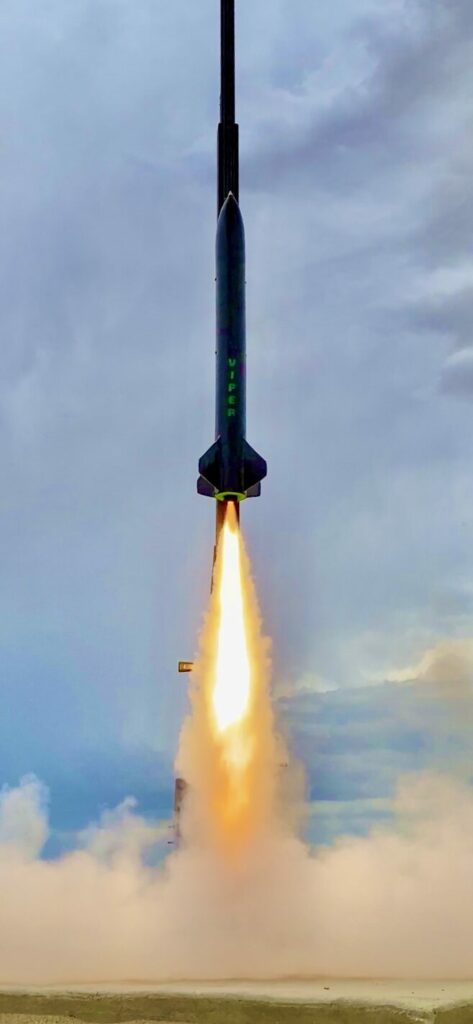
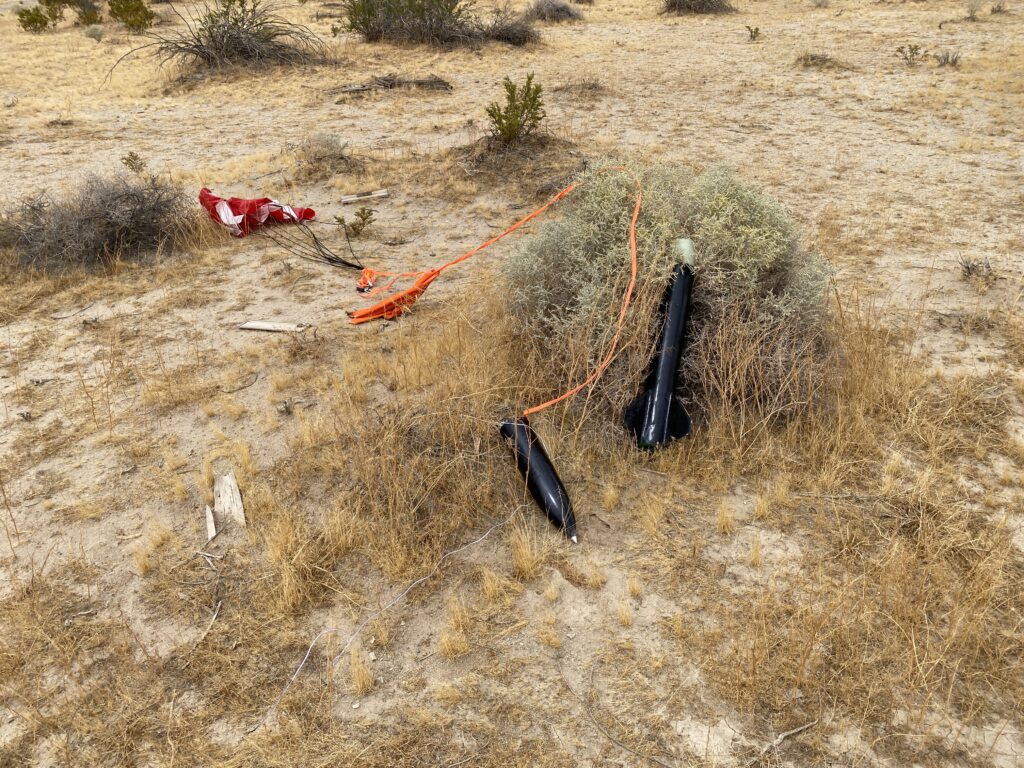
Article:
I decided to build the same rocket for my L1 and L2 certification flights. Viper is a 4.25″ OD, 4″ ID rocket with 4 trapezoidal NACA 0015 fins, a 9.5″ parabolic nosecone, and a 38mm motor tube. It uses a 48″ parachute with a Cd of 1.5, landing at 19 fps, and should have an apogee of 905 feet on the L1 flight and 2,846 feet on the L2 flight.
Viper was launched at FAR (Friends of Amateur Rocketry) in the Mojave Desert on August 5th, 2023 off of a 10-foot rail. It launched again on August 19th, 2023, off a 20-foot rail for my L2 certification. It uses redundant electronic and motor ejection systems to ensure recovery success and has a single main parachute.
Viper is constructed of two materials: fiberglass and 3D-printed ABS. I originally used PLA to 3D print the motor centering rings, recovery bulkhead, tracker stand, and fin/nosecone molds. However, after some tests, I became concerned with PLA’s poor heat tolerance. Considering the Mojave can often exceed 110 degrees ambient, I decided to reprint the centering rings and recovery bulkhead from ABS, which is less rigid but has excellent heat tolerance.
For the body tube, nosecone, and fins I laid a single piece of fiberglass. While this means compromising on modularity for future upgrades of Viper, I made this decision to ensure that the entire vehicle was completely rigid, especially since the fins were surface mounted.
The first step was designing the vehicle in Open Rocket. Since it needs to be stable for both I and J motors, I had to consider both platforms in the simulations. I also wanted the vehicle to be stable at supersonic speeds since, once I secure my L2 certification, it might be fun to throw in a K motor and launch it to Mach 1.15.
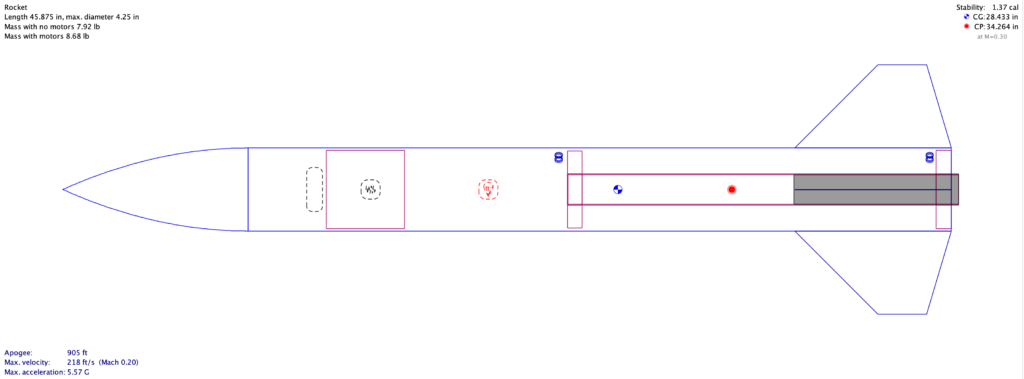
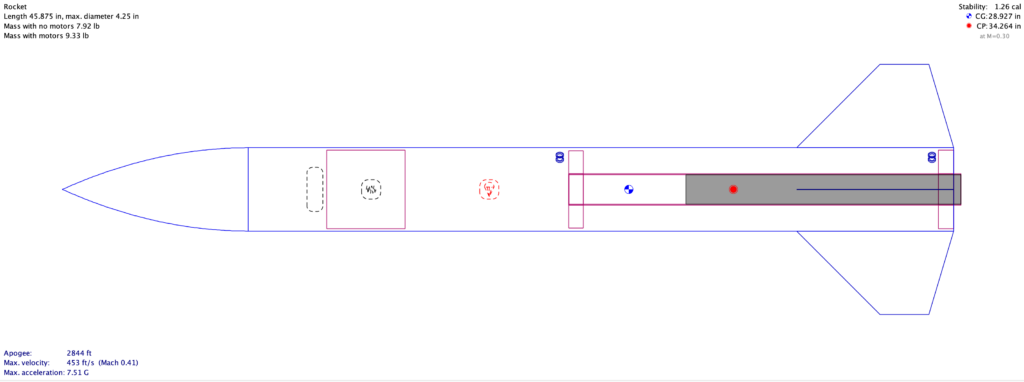
The fins are trapezoids, with a root chord of 8″, tip chord of 2.5″, height of 4.25″, and sweep of 45 degrees. They are based on a NACA 0015 airfoil and taper along its chord. Since this project was my first time laying up fins in fiberglass, I didn’t want to overcomplicate the design, so I stuck with a strong and simple design that would also improve the aerodynamics.
Since I have aspirations of one day flying it on a K/L motor, I made the fins 0.25″ thick on either side to prevent fin flutter. According to my calculations, fin flutter would begin at 1,628 mph (Mach 2.12) so the fins would be more than strong enough for any conceivable flight. Furthermore, the fins would be sturdy so that, during recovery, they wouldn’t snap upon impact with the ground.
The next step was creating the CAD files for the motor centering rings and recovery rings in SolidWorks. Since I wanted to one day reuse this airframe with a 54mm motor system, I decided to make the motor tube swappable. Typically, model rockets of this size use plywood centering rings that are epoxied to the cardboard airframe. However, since I was using 0.125″ thick fiberglass walls, I opted for using 4 1/4-20 bolts to anchor the motor since it would give me the modularity I sought.
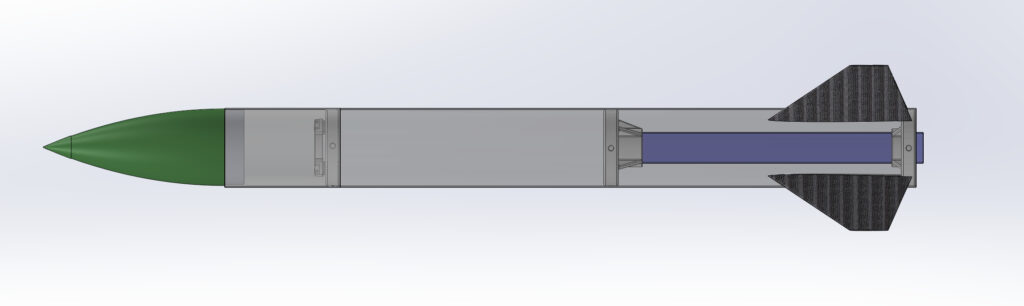
Because I only had access to a 3-D printer, I developed my own design for interfacing the motor rings with the 1/4-20 bolts. Using traditional bolts with a free nut would’ve proved extremely challenging for vehicle assembly, so instead I included a custom recess for the nut, and then used JB weld to ensure adhesion. That way, ideally, I could simply screw in eight bolts from the outside and the motor assembly would be anchored in place. In reality, this proved to be somewhat problematic, since the force of screwing in the bolts unseated the nut several times, so I had to reinforce it using CA glue.
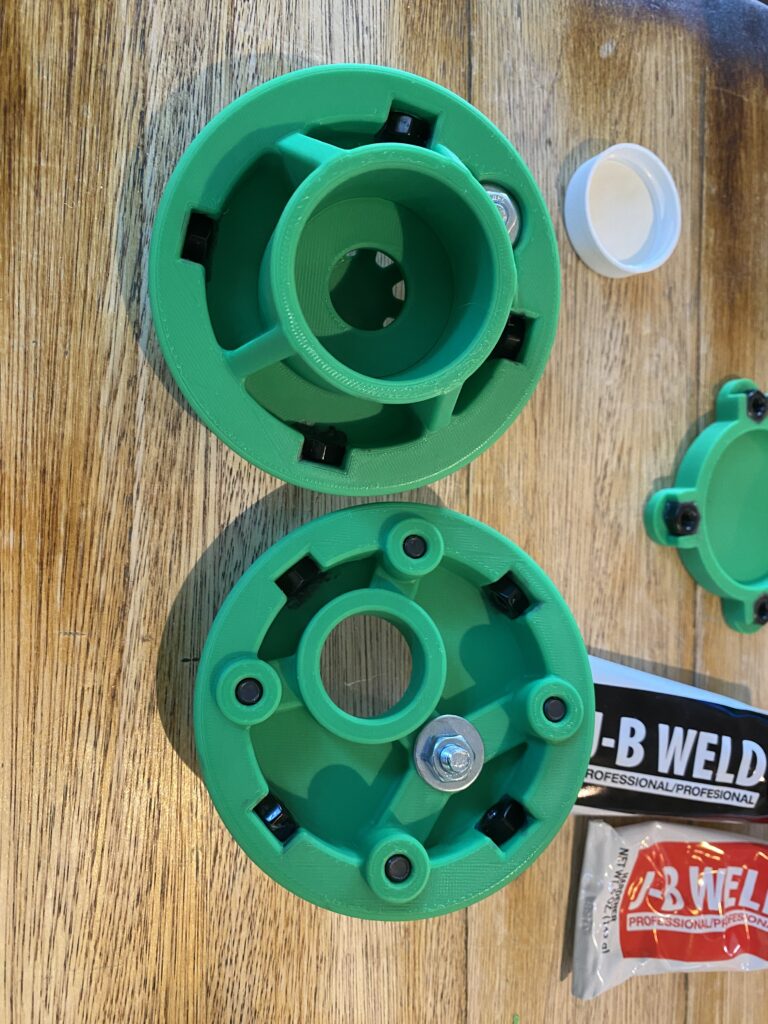
Another feature I wanted to include was a gas baffle. During my research for this project, several experienced builders reported problems with the hot gas and solid particles ejected from the motor recovery deployment charge damaging the parachute. To prevent this, I designed a baffle in the upper motor centering ring. The baffle works by providing an impedance to the hot black powder particles but still providing a pathway for the gas to eject the parachute.
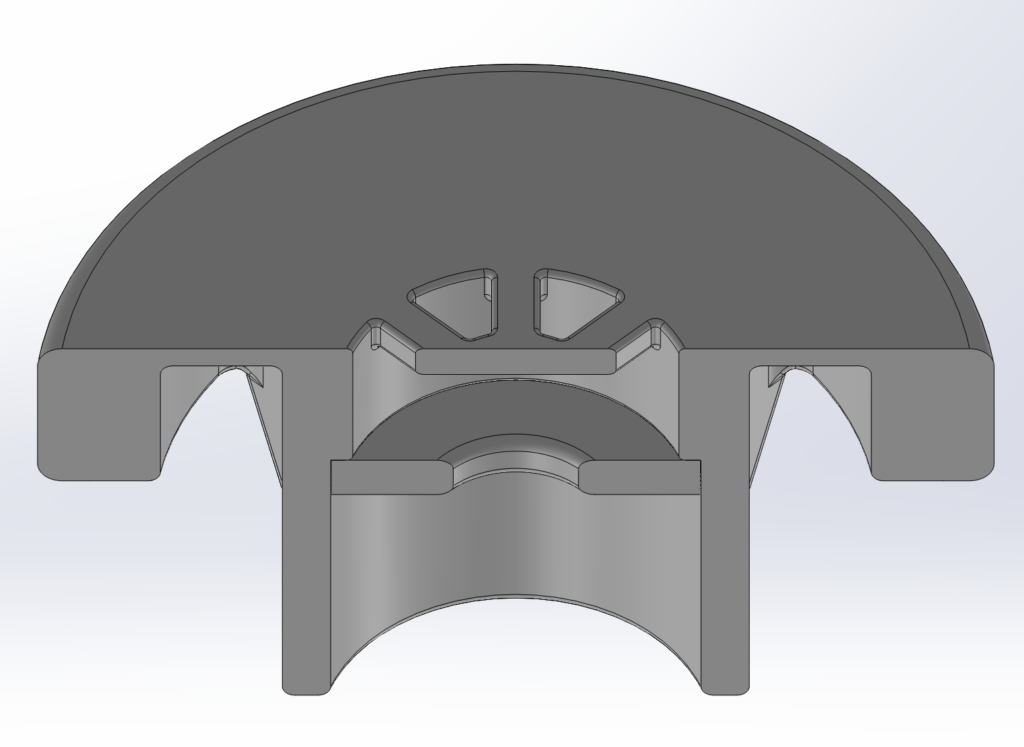
Once the designs were finalized, the rings were printed out of PLA using a 50% infill for rigidity.
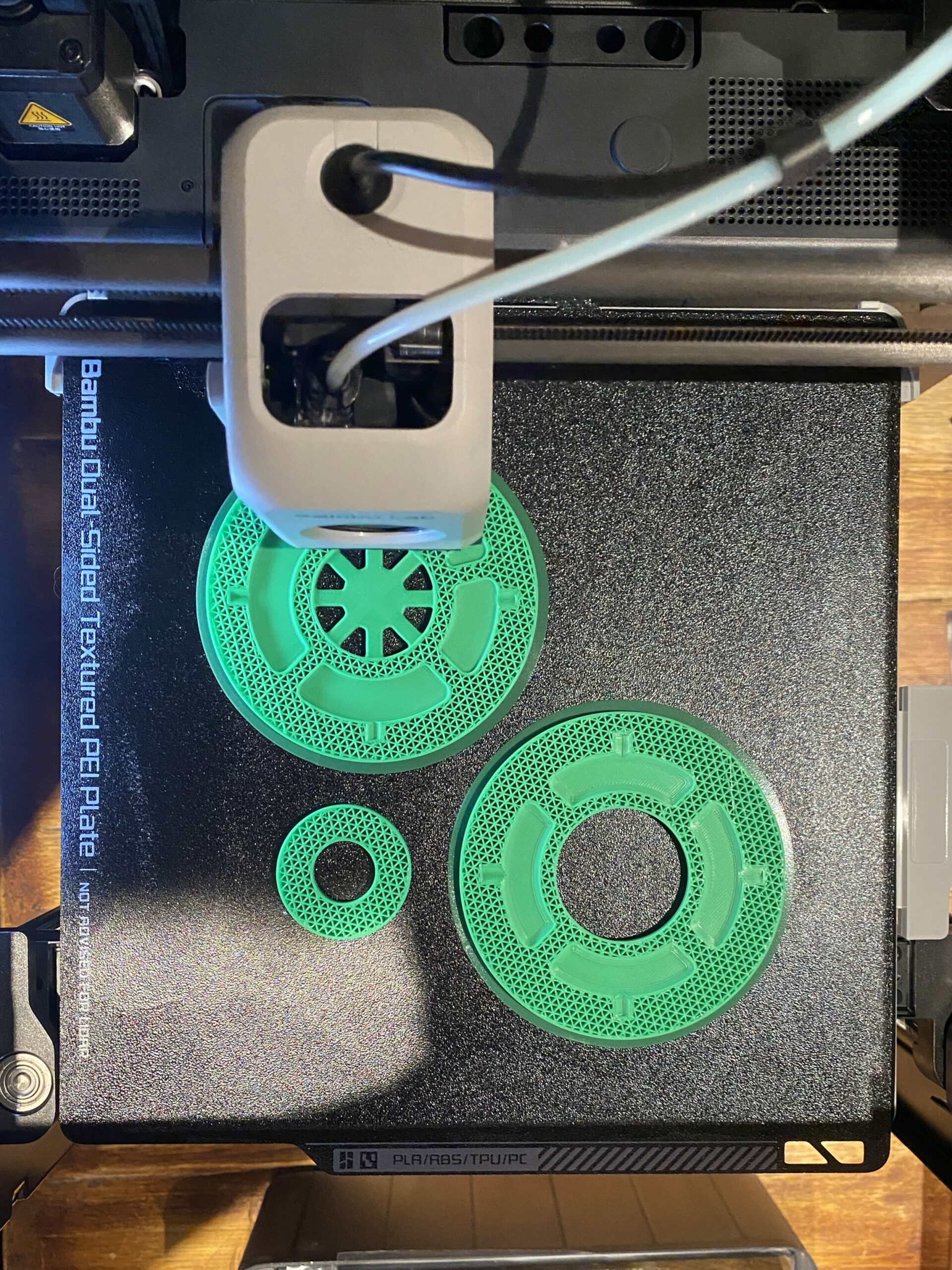
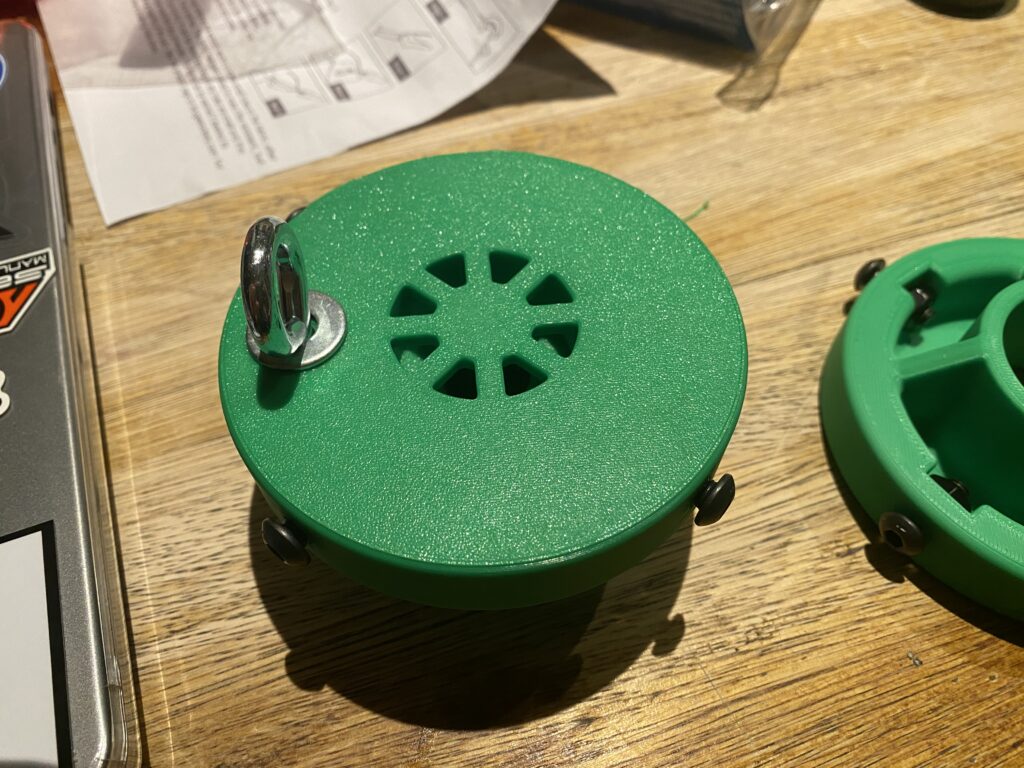
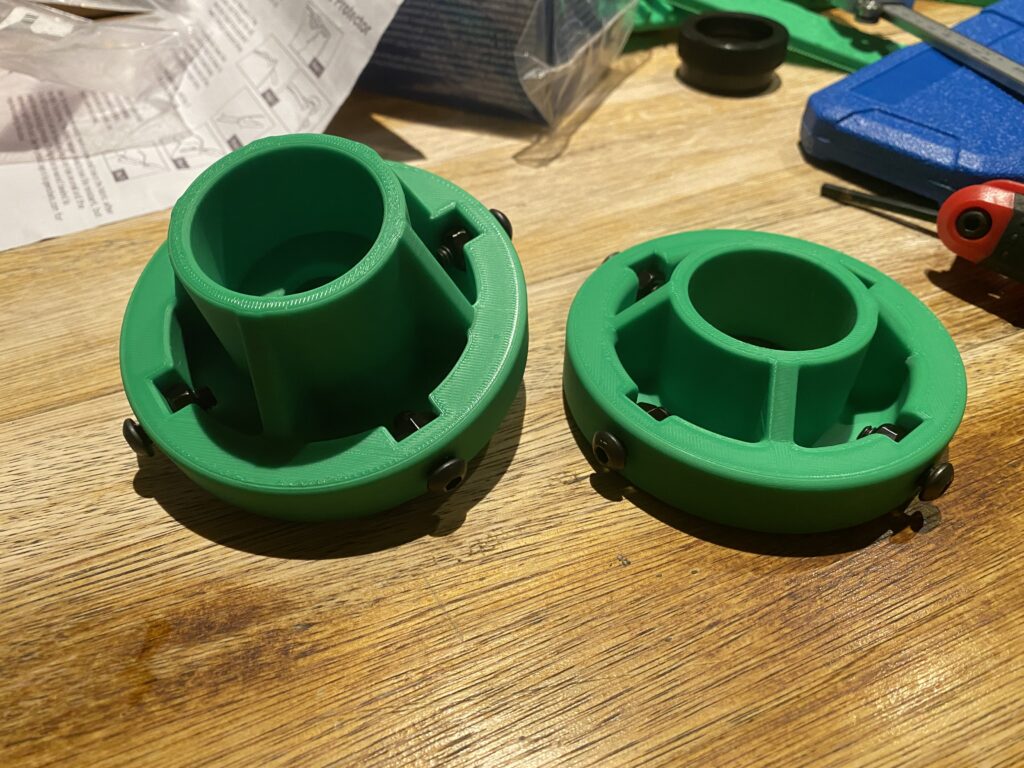
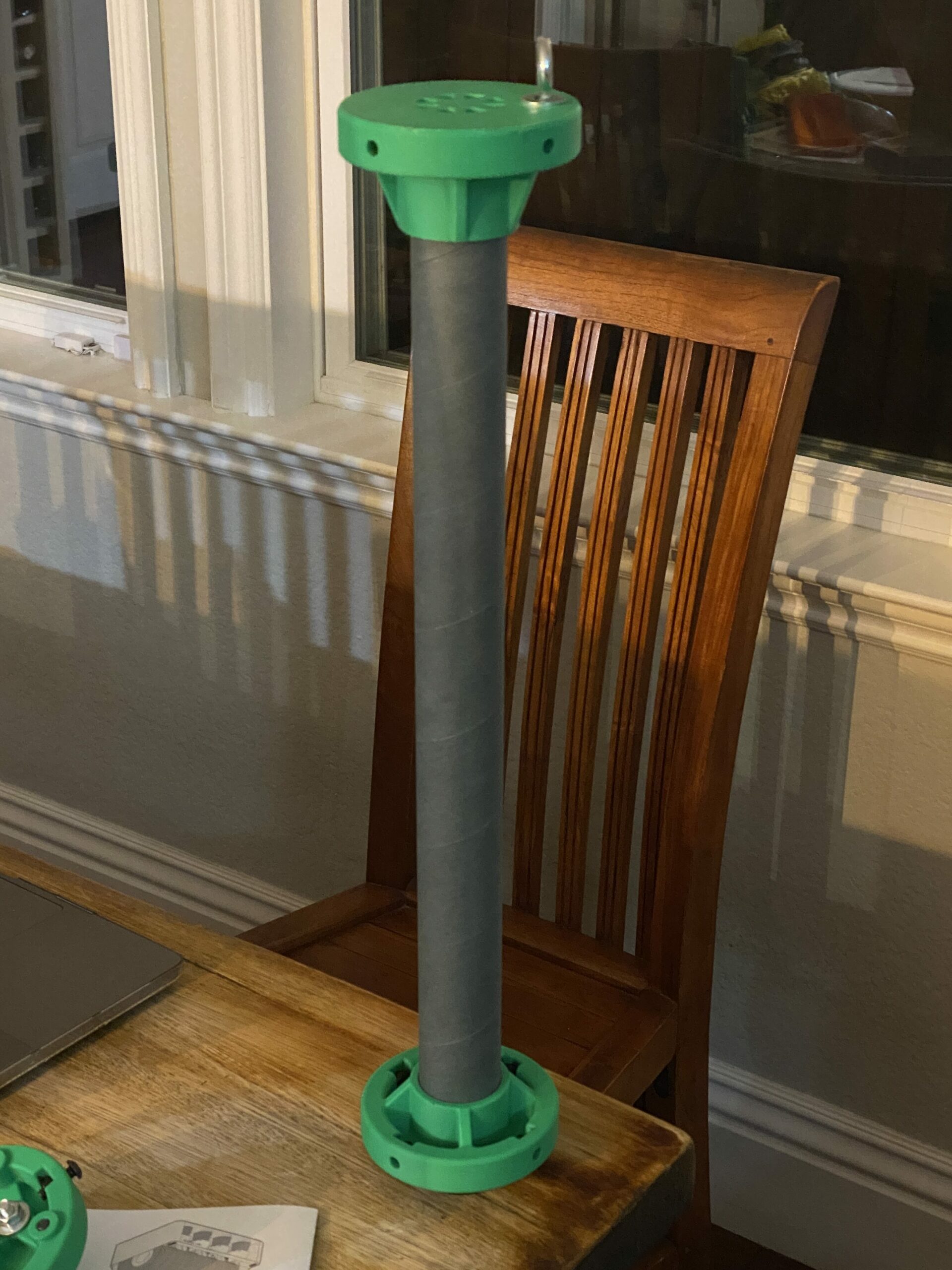
The motor tube interfaces with the airframe using 4 1/4-20 bolts. I epoxied nuts in the slots in the centering rings for securing the bolts. Based on my shear-out calculations, the factor of safety of 1/4-20 bolts in 1/8″ thick fiberglass was 5x greater than even the G-force sustained under a K motor, so I was confident this solution would be strong enough.
Next step: fins. I opted to use a 3D-printed mold for the fins that would remain in the final product for ease of manufacturing. I developed my own fin can jig to hold the fins in the precise position and ensure alignment
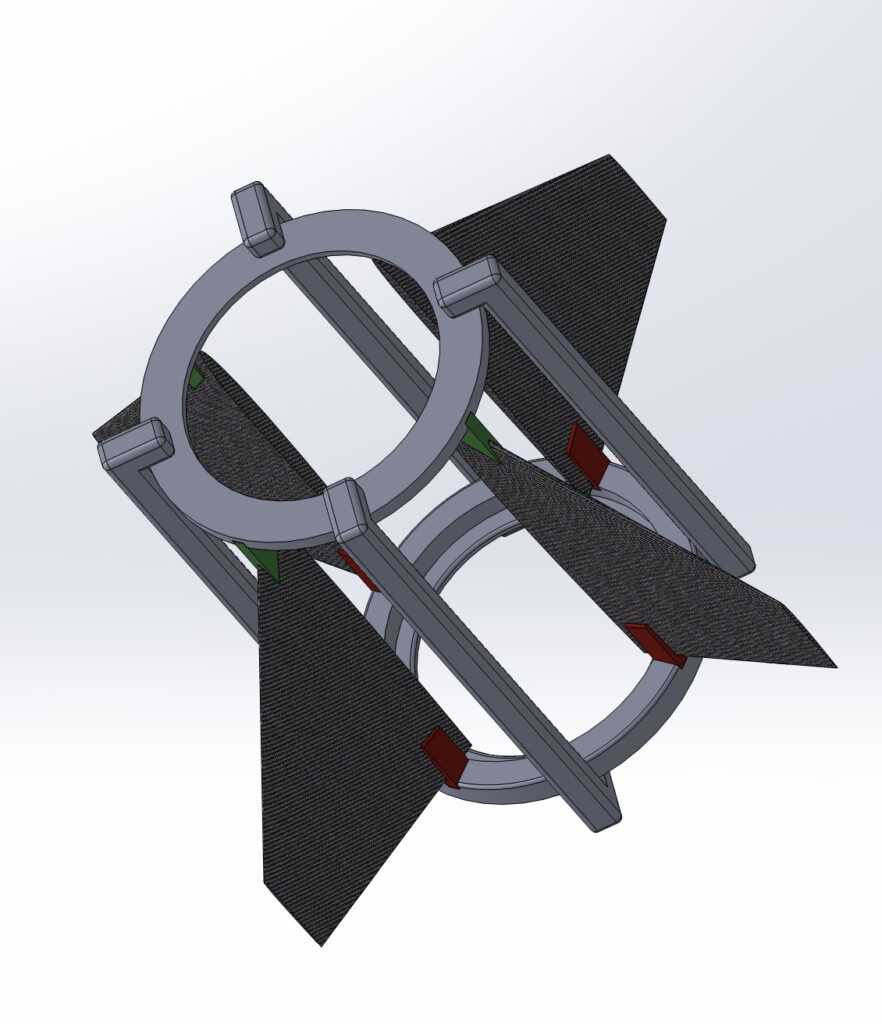
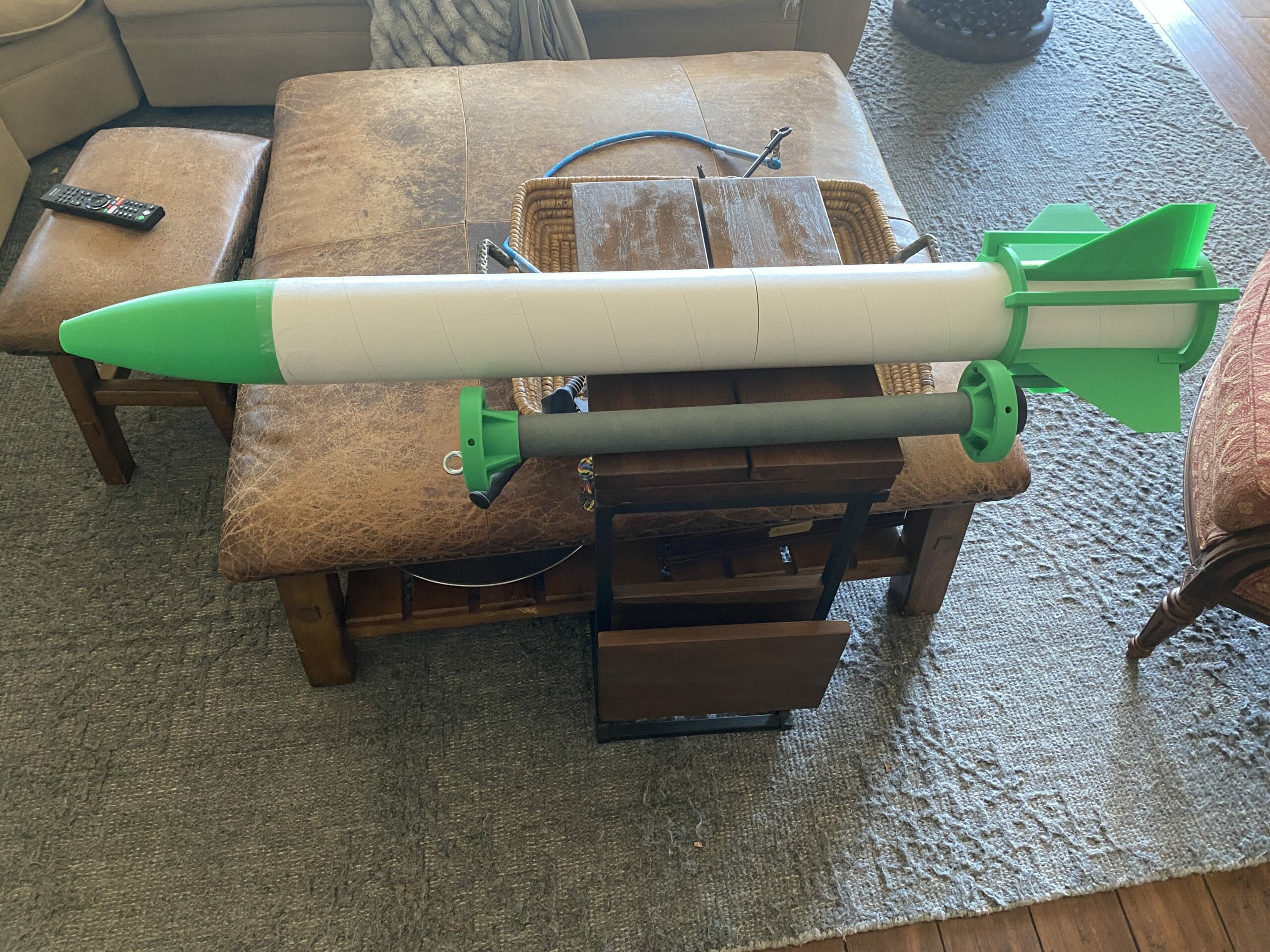
For the fiberglass layups, I decided to use 6oz fiberglass cloth with epoxy resin from Total Boat. I used 8 layers of fiberglass on all surfaces. One mistake I made was not using the established nosecone manufacturing method of laying up the fiberglass cloth in a clamshell-like mold. Instead, I wrapped it in small strips of cloth. The end result was that the surface finish was inconsistent and to achieve a smooth profile I had to spend hours upon hours adding filler and sanding.
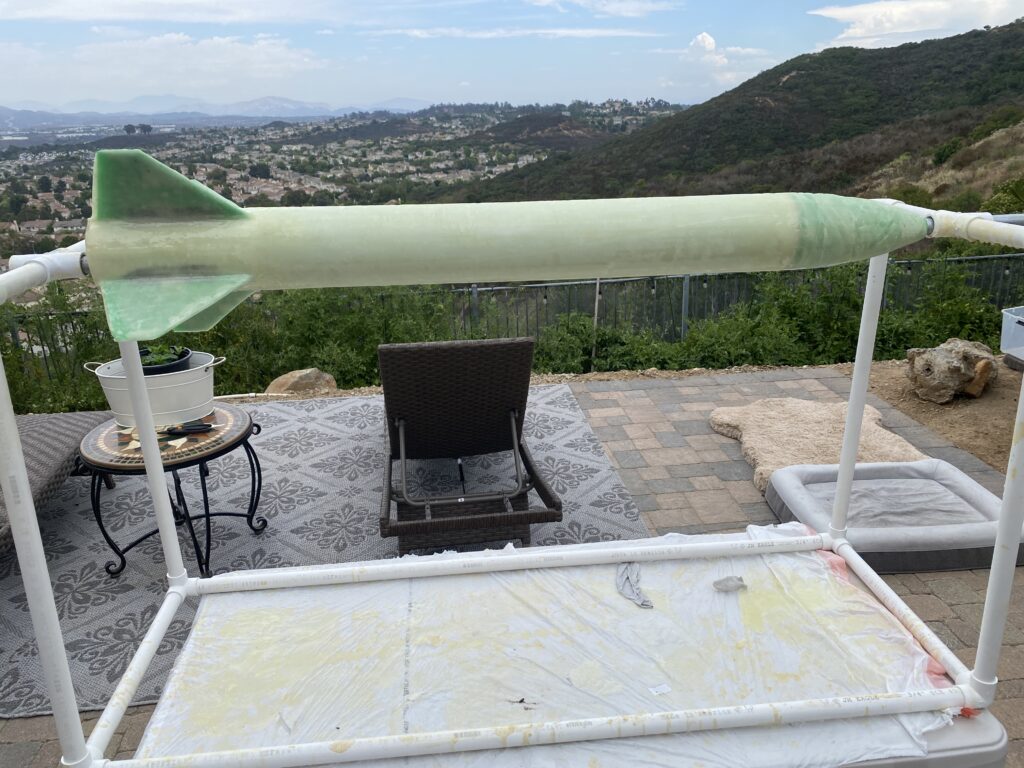
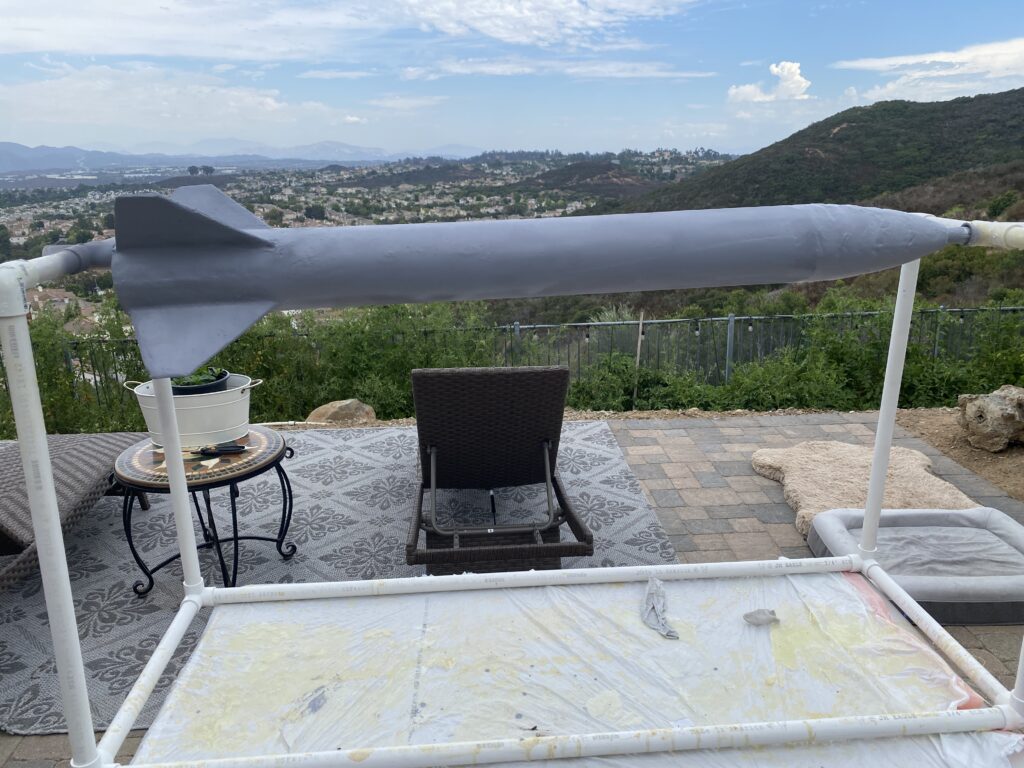
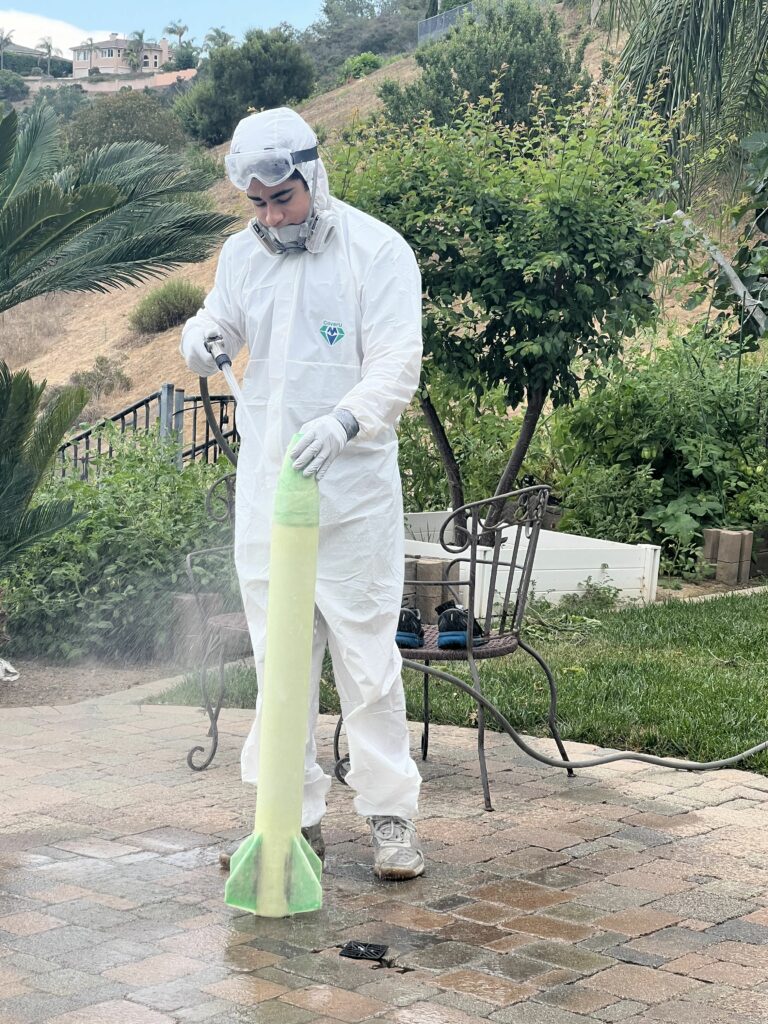
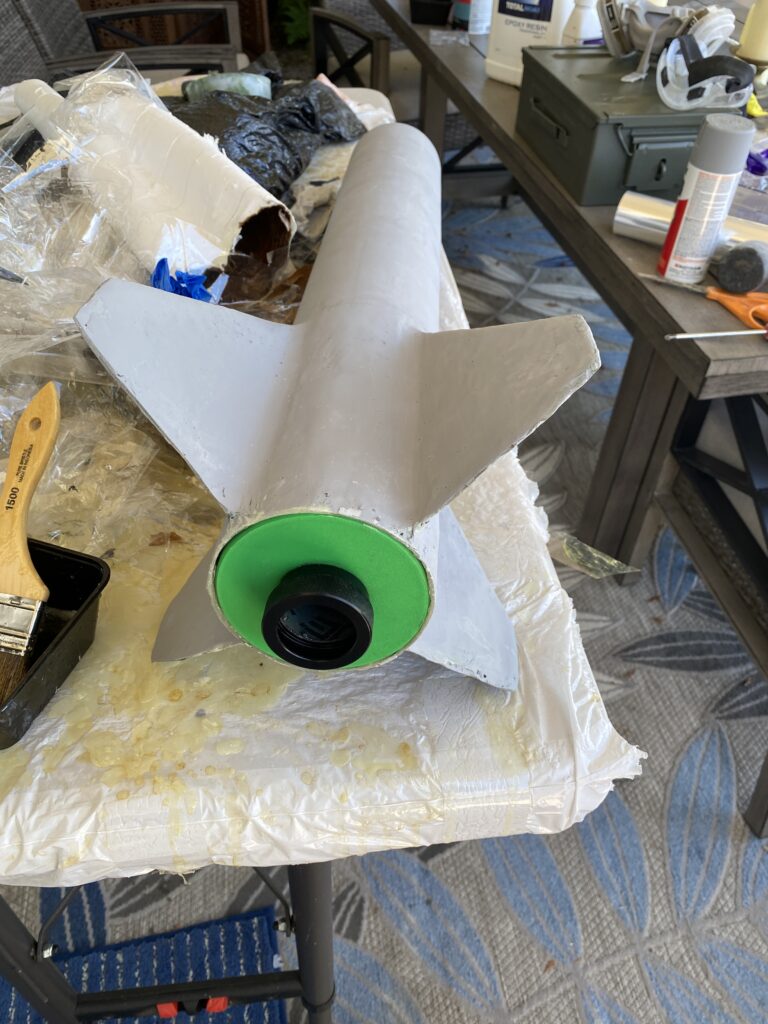
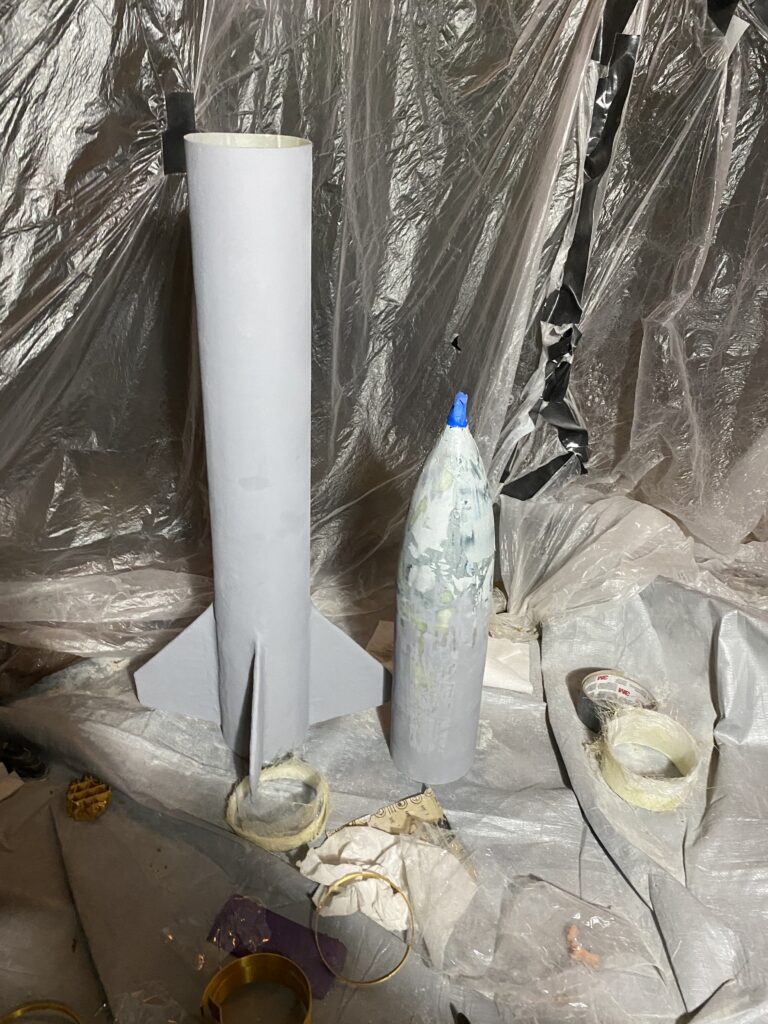
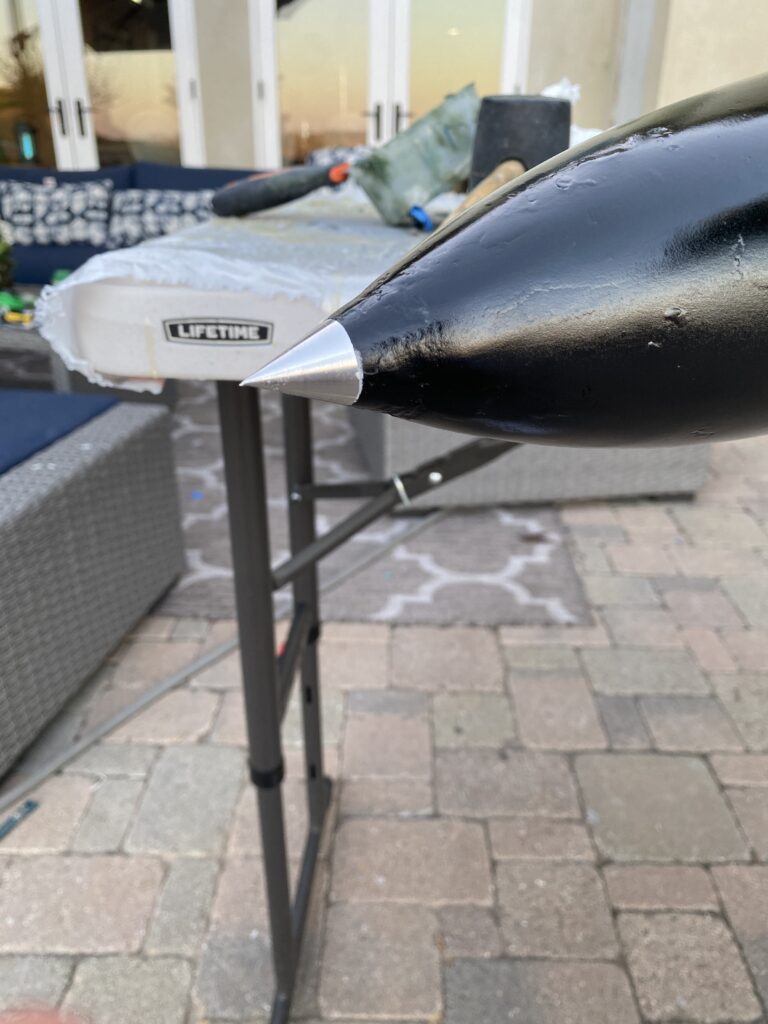
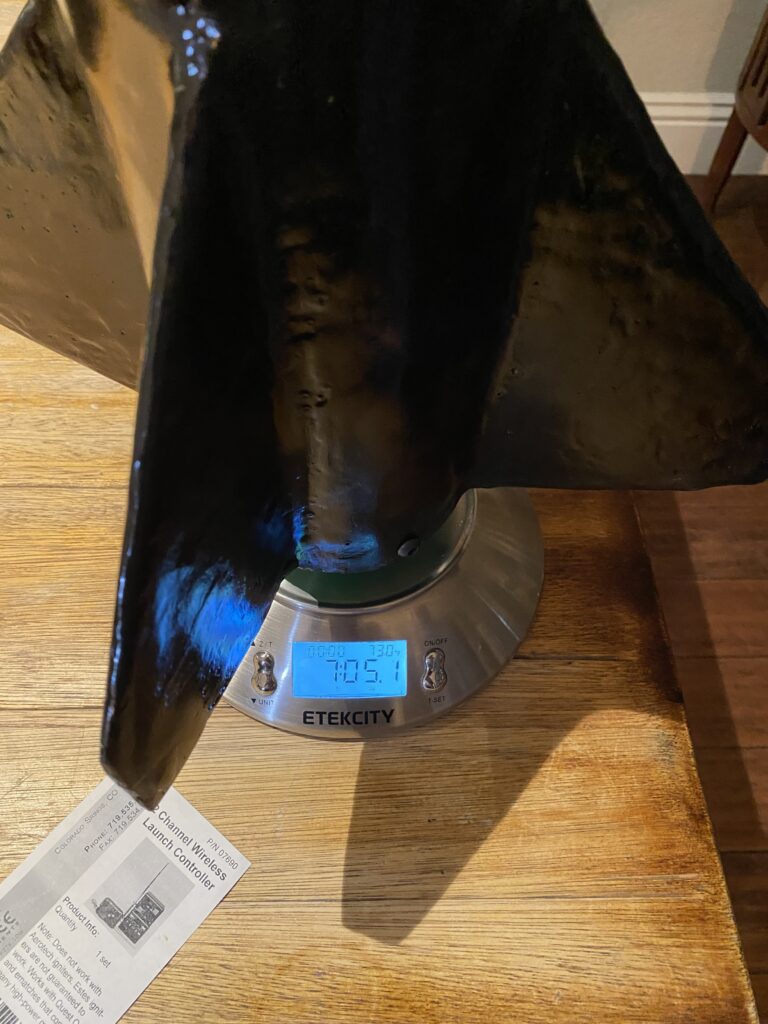
I forgot to take photos of the recovery setup, but I used a 48″ elliptical parachute and 30ft tubular nylon shock cord. About 2/3 of the way up the shock cord, I tied a butterfly knot to attach the parachute. I also manufactured a fiberglass coupler tube that fits between the two halves. Ensuring a snug fit was a challenge, but I used a bit of lubrication to ensure it wasn’t so tight that it prevented recovery deployment.
I originally planned to use redundant electronic recovery using an Eggtimer Apogee, but my soldering skills were not up to the intricate kit and I could not get it working in time for launch, so I relied solely on the motor ejection deployment timed based on my Open Rocket simulations.
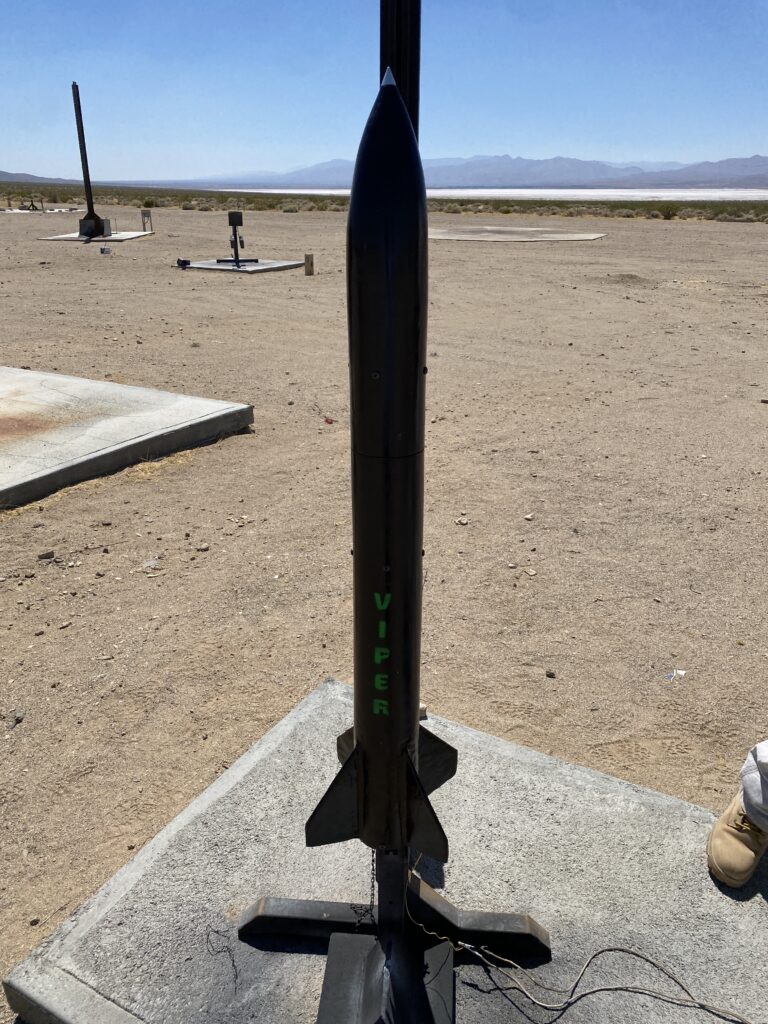
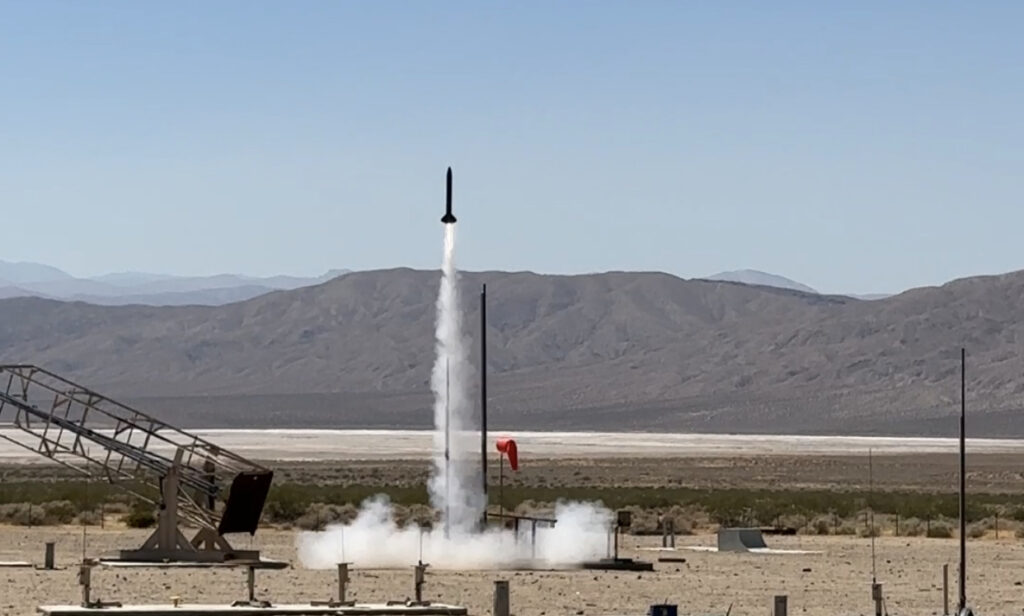
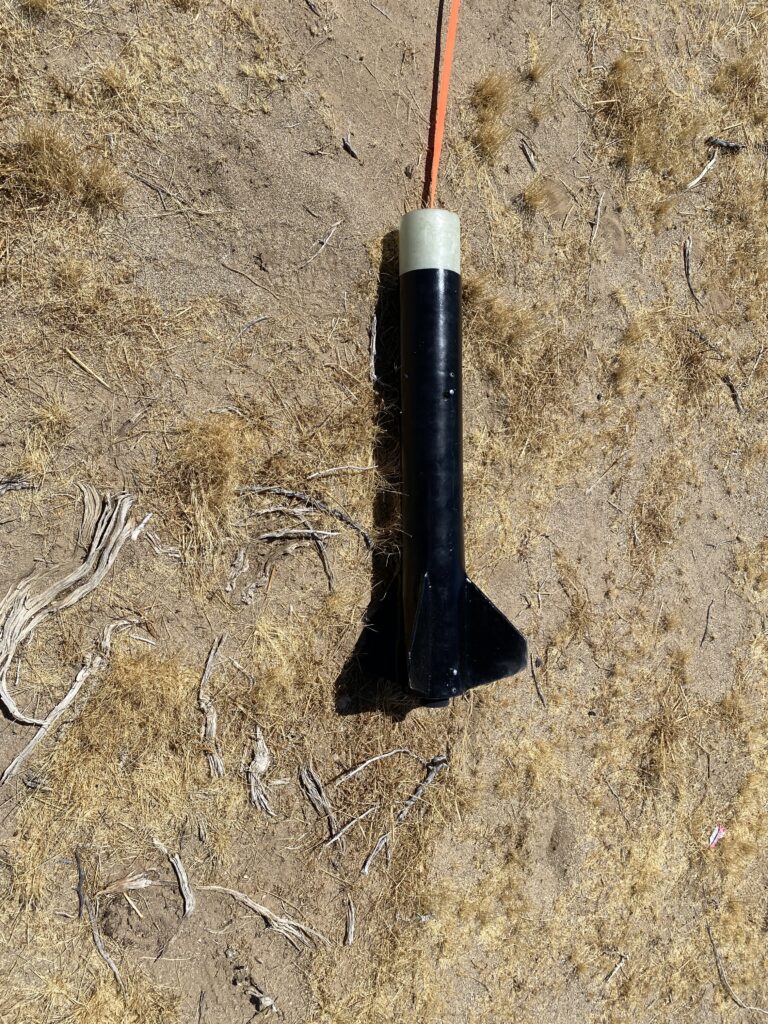
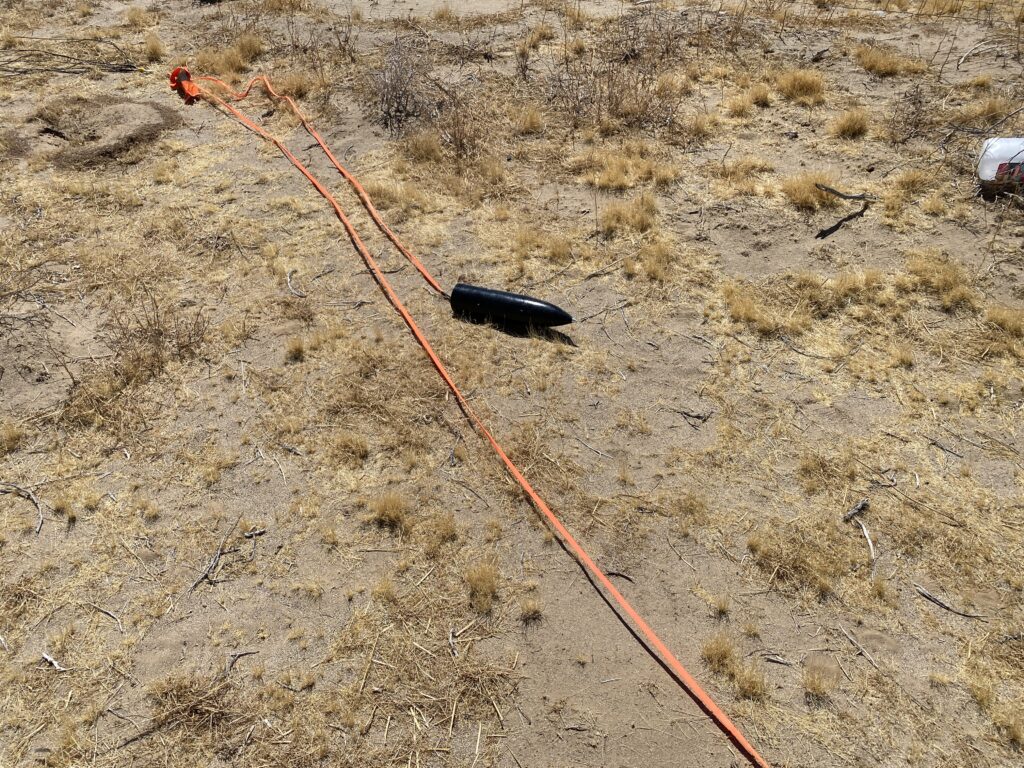
Unfortunately, the ejection gas baffle and “fireproof” parachute protector were both unable to prevent the hot gas from burning a hole through the chute. Thus, the parachute won’t be reusable for a future flight. However, the shock cord was undamaged thankfully.
To achieve my objectives of L1 and L2 certification for the summer, I had two weeks of turnaround to prepare for the L2 flight after my L1 cert. Other than preparing for the exam, I also wanted to include redundant electronic recovery deployment.
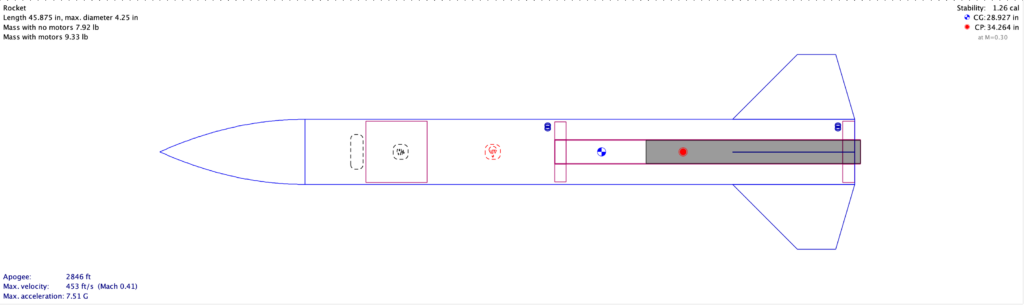
I originally experimented with using an Eggtimer Apogee kit. This was attractive because it was relatively simple and lightweight, not to mention cheap, but it required soldering, a weakness of mine.
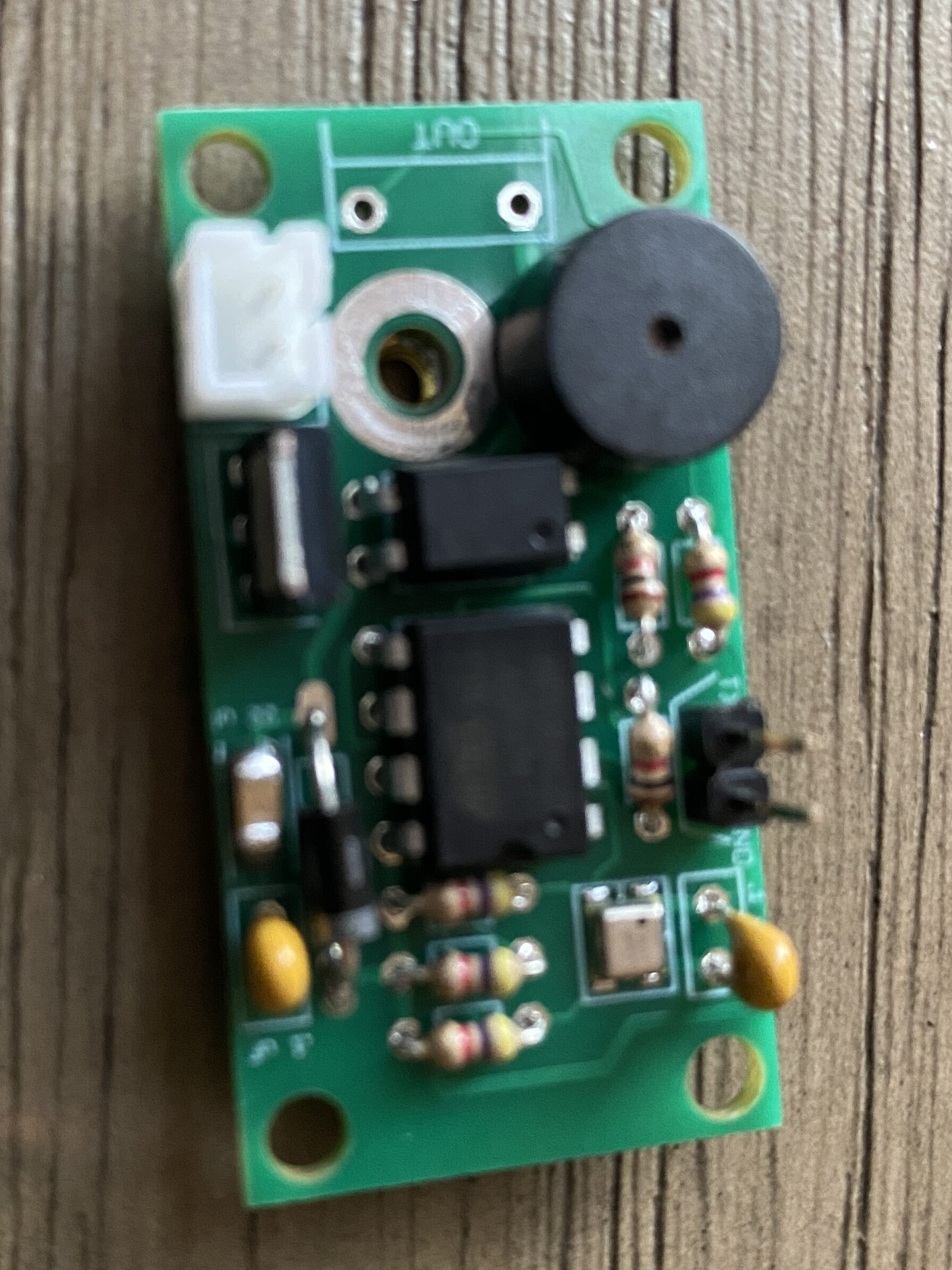
Since the Eggtimer Apogee was a failure, I opted instead for just purchasing a standard dual deployment altimeter, a Missileworks RRC3, that I could grow into with future projects (it then happened to fly on our 15′ hybrid rocket at FAR-OUT as a redundant deployment altimeter). I also decided to strip down a basic commercial car GPS tracker, although it didn’t end up working on the flight and led to a long walk through the desert.
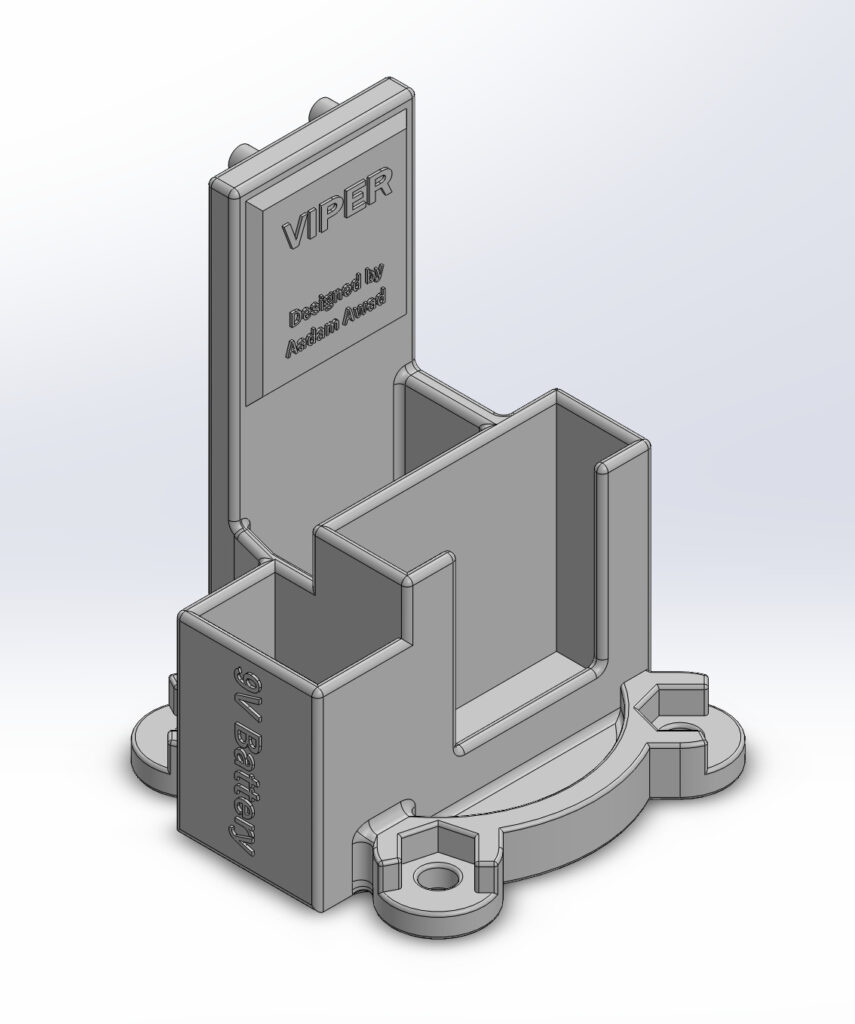
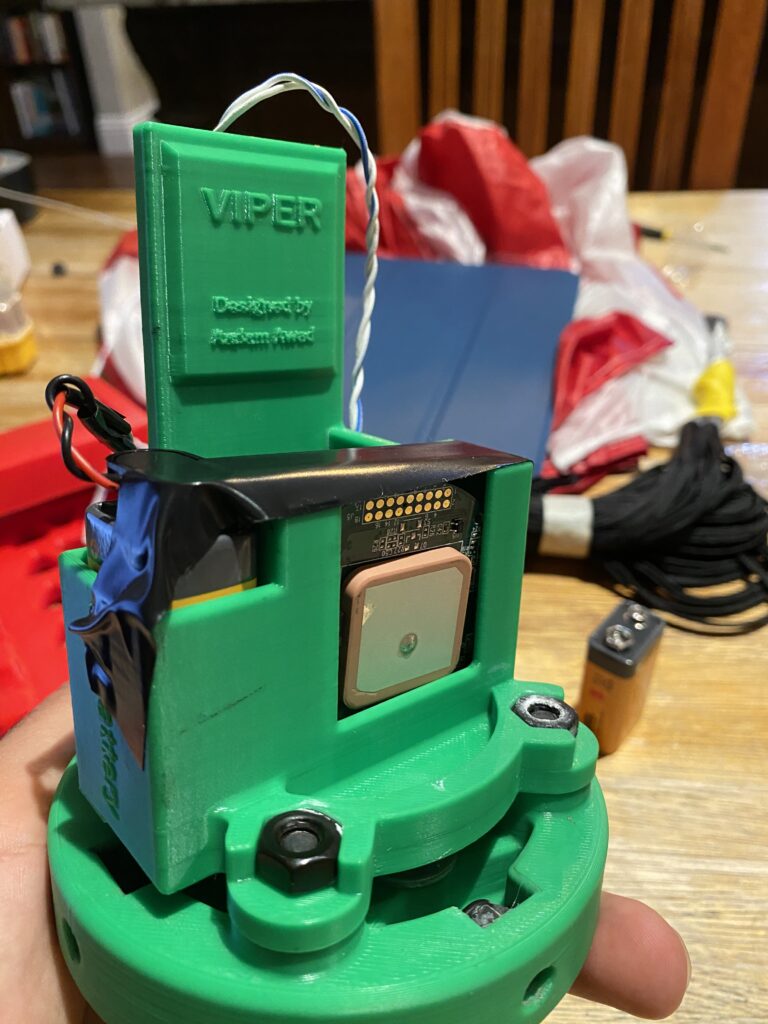
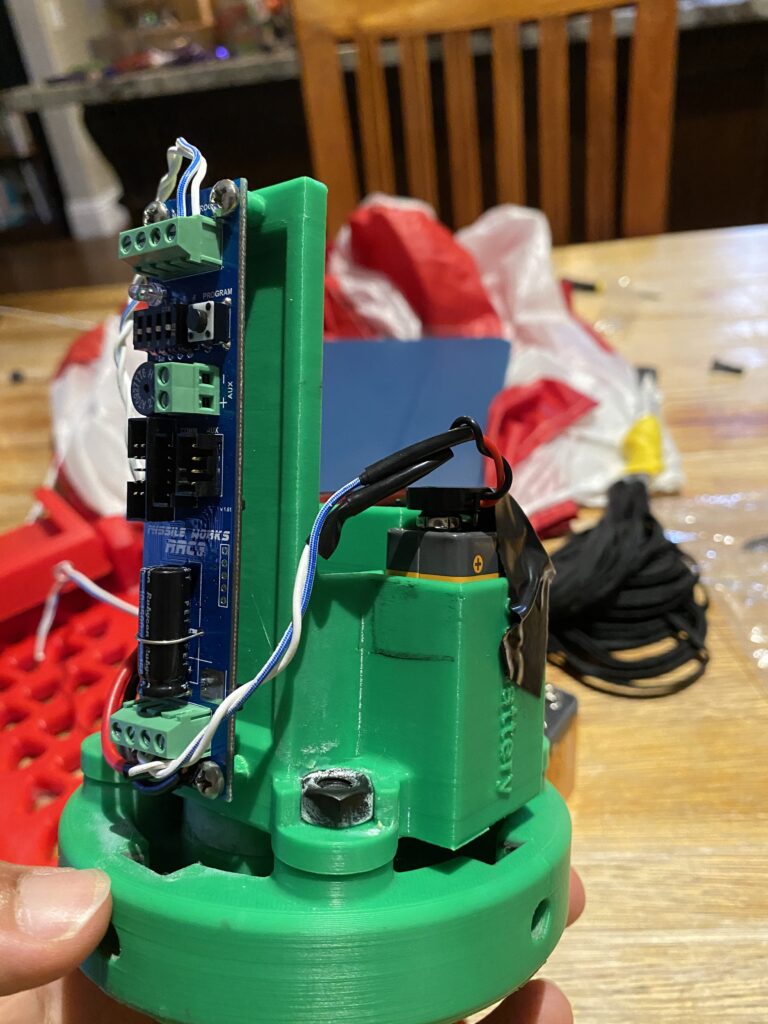
For the electronic deployment, I only wired up the e-match to the drogue terminal. In a dual-deployment setup, the drogue parachute should be fired at apogee. Then, once the barometer detects the vehicle has reached some pre-programmed altitude (like 300 or 500 feet) then the main parachute will fire and the vehicle can drift down to the ground. Since Viper is only designed to use a single main parachute, only the drogue terminal is needed.
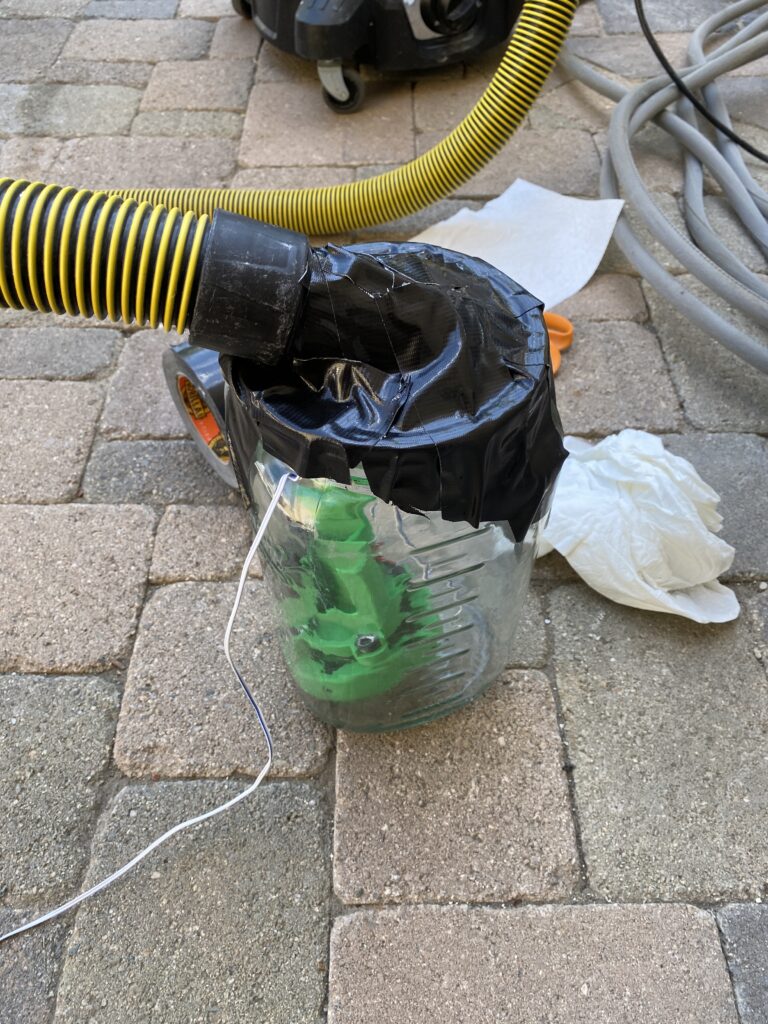
Once all the new hardware was fully tested, I prepared the vehicle for flight and made the trek back up to FAR for my L2 certification attempt. After getting 40/40 on the L2 exam, it was time to fly. I was nervous because Southern California was being hit with the first tropical storm in a century, but fortunately this was a low apogee flight.
For my L1 flight, I used 1/4″ rail buttons for 1010 rails. However, Rick Maschek encouraged me to use the larger 1015 rail buttons for when I returned to FAR for my L2. On my second trip, I was extremely glad of this change since it enabled me to use a 20′ rail, which was definitely necessary considering the wind speeds.
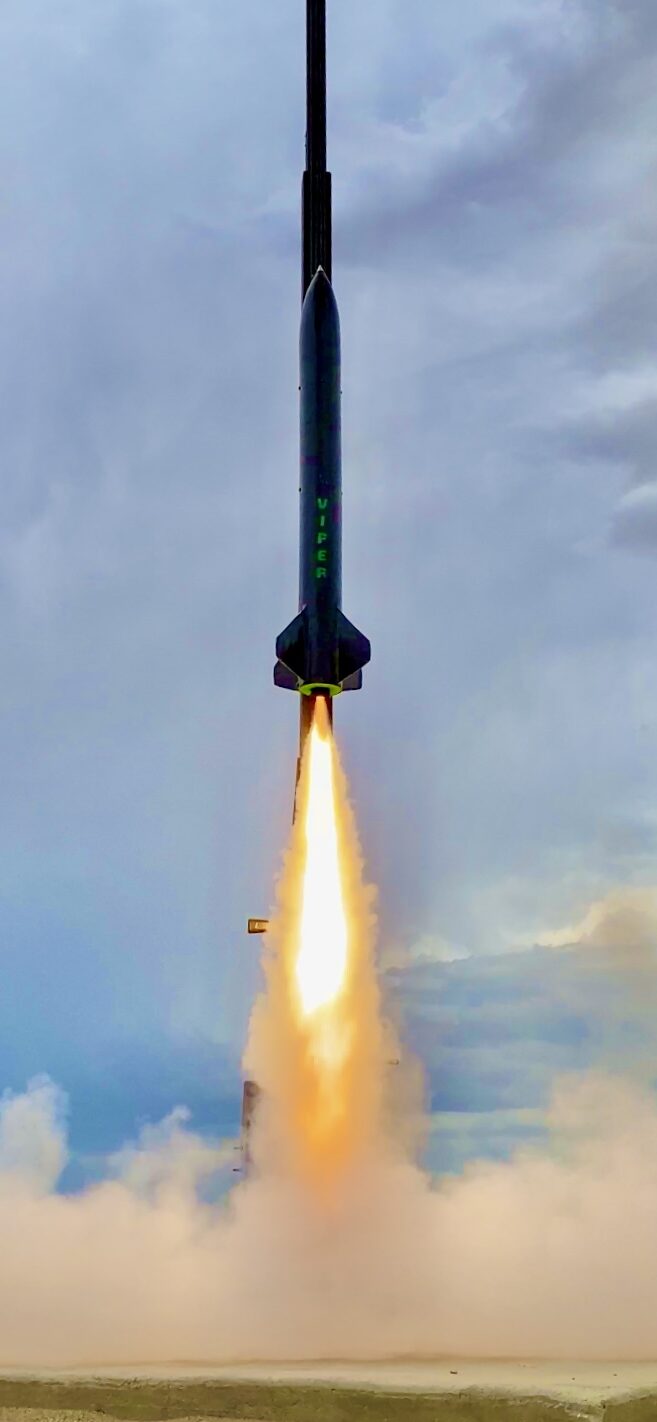
Since Viper only had a single-stage recovery chute, it drifted quite a lot down range. My GPS malfunctioned so the only guidance we had for recovery was a line in the dirt and a video. After setting out with some water and a healthy dose of optimism, we combed through the desert. About an hour and a half later, I found the rocket tangled in some brush!
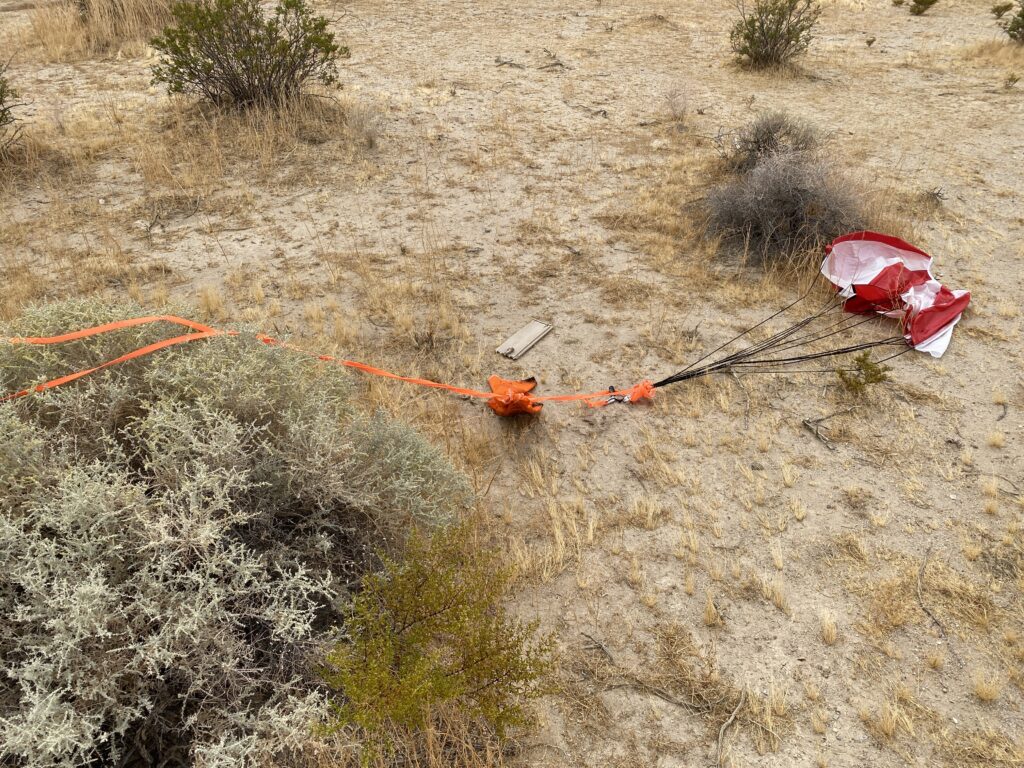
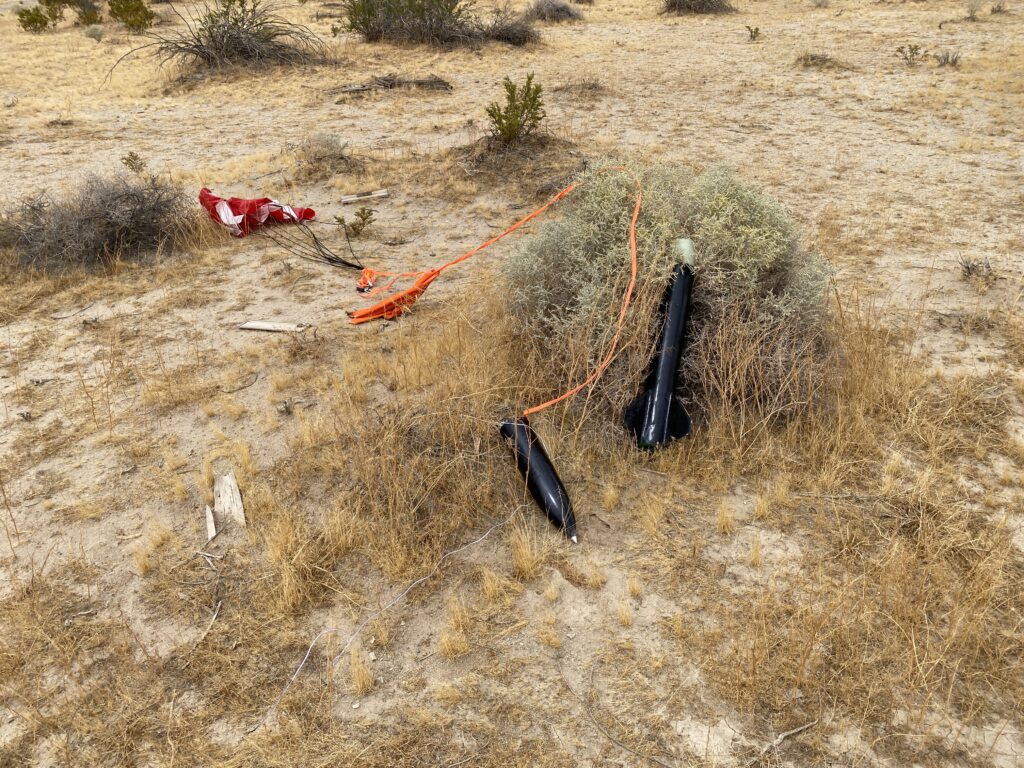
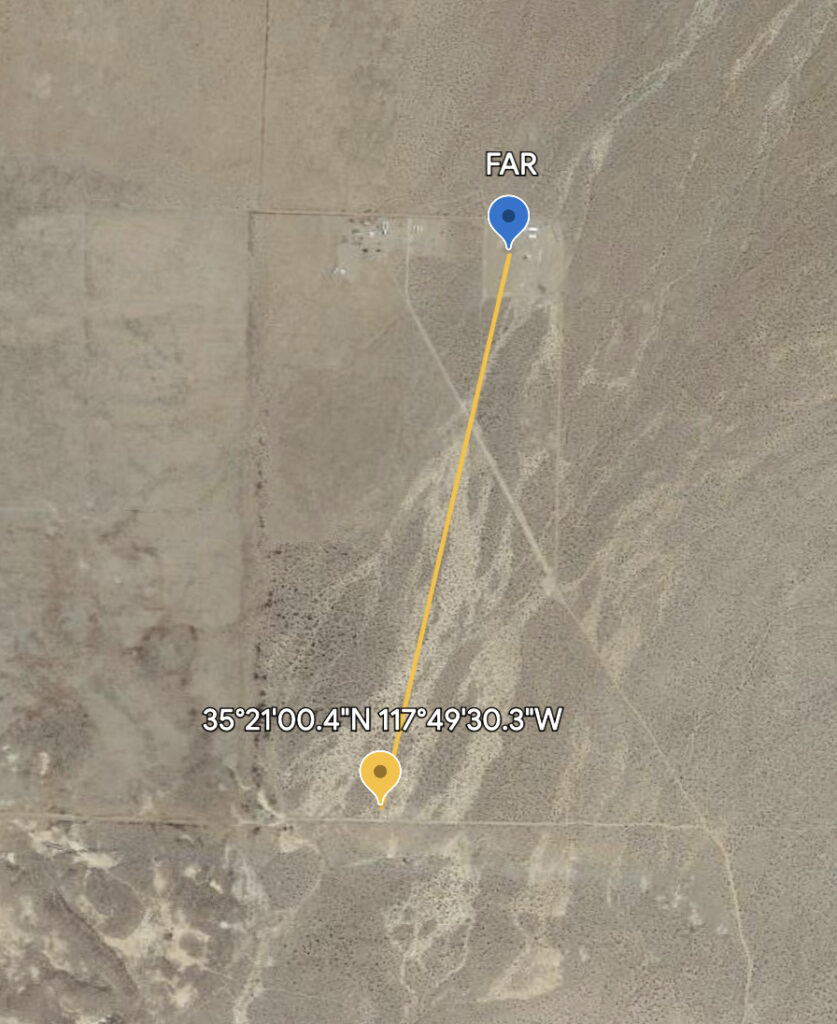
Overall, this was one of the most rewarding projects I’ve pursued. Throughout the process of building and flying Viper, I learned about composites, rocketry principles, electronics, solid rocket motors, and aerodynamics. As I continue to work on our nitrous oxide system for the CSI hybrid rocket, I’ll brainstorm for a new rocket to build in the future.